Abstract
In recent years, the Yanchang shale-oil formations of the Ordos Basin are rich in reserves with complex lithology and structure characteristics, low porosity and low permeability, and weak anomalies for oil and water discriminations, have been the key targets of unconventional oil/gas resource exploration and development in the relevant areas. The joint acoustic-electrical (AE) properties can be used to interpret reservoir lithology, mineralogy, pore structure, and fluid saturation. To conduct tests of thin section analysis, X-ray diffraction, and ultrasonic and electrical experiments at different pressures and saturation degrees, cores from the shale-oil formations in the Q area of the basin are collected. The variations in AE properties with respect to clay content, porosity, pressure (microfracture), and saturation are analyzed. The experimental results indicate that the rock physics behaviors of sandstones with different clay contents vary significantly. The AE properties of clean sandstones are basically dependent on the microfractures (pressure), while for muddy sandstones, the clay content is an important factor affecting the responses. The target reservoir consists of interbedded sandstone and shale layers. The AE equivalent medium equations and the Gurevich theory are applied to establish the joint models for the different lithologies and simulate the variations in AE properties with respect to fluid type, pore structure, and mineral components. The three-dimensional joint templates of clean and muddy sandstones, as well as shale, are developed based on the elastic and electrical attributes and then calibrated using the experimental and well-log data. The reservoir properties are estimated with the templates and validated by the log data. The results indicate that the joint templates based on lithology characteristics can effectively characterize the properties of interbedded sandstone and shale layers. Furthermore, the combined application of AE data provides more beneficial information for the assessment of rock properties, leading to precise estimates that conform with the actual formation conditions.
Similar content being viewed by others
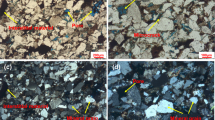
References
Aguilera, M. S., and Aguilera, R., 2003, Improved models for petrophysical analysis of dual porosity reservoirs: Petrophysics, 44(01), 21–35.
Amalokwu, K., Best, I.A., Sothcott, J., et al., 2014, Water saturation effects on elastic wave attenuation in porous rocks with aligned fractures: Geophysical Journal International, 197(2), 943–947.
Archie, G., 1942, The electrical resistivity log as an aid in determining some reservoir characteristics: Transactions of the AIME, 146(1), 54–62, doi: https://doi.org/10.2118/942054-G.
Asami, K., 2002, Characterization of heterogeneous systems by dielectric spectroscopy: Progress in Polymer Science, 27(8), 1617–1659.
Ba, J., Xu, W., Fu, L., et al., 2017, Rock anelasticity due to patchy-saturation and fabric heterogeneity. A double-double porosity model of wave propagation: Journal of Geophysical Research: Solid Earth, 122(3), 1949–1976, doi: https://doi.org/10.1002/2016JB013882.
Batzle, M., and Wang, Z., 1992, Seismic properties of pore fluids: Geophysics, 57(11): 1396–1408.
Berryman, J. G., 1980, Long-wavelength propagation in composite elastic media: Journal of Acoustical Society of America, 68(6), 1809–1831.
Berryman, J. G., 1992, Single-scattering approximations for coefficients in Biot’s equations of poroelasticity: Acoustical Society of America Journal, 91(2), 551–571.
Berryman, J. G., 1995, Mixture theories for rock properties. Rock physics phase relation: A Handbook Physics Constants, 3, 205–228.
Chapman, S., Tisato, N., Quintal, B., et al., 2016, Seismic attenuation in partially saturated Berea sandstone submitted to a range of confining pressures: Journal of Geophysical Research: Solid Earth, 121(3), 1664–1676.
Cilli, P., and Chapman, M., 2021, Linking elastic and electrical properties of rocks using cross-property DEM: Geophysical Journal International, 225(3), 1812–1823.
David, E. C., and Zimmerman, R. W., 2012, Pore structure model for elastic wave velocities in fluid-saturated sandstones: Journal of Geophysical Research: Solid Earth, 117(B7), B07210.
Dutilleul, J., Bourlange, S., Géraud, Y., et al., 2020, Porosity, pore structure, and fluid distribution in the sediments entering the northern Hikurangi margin, New Zealand: Journal of Geophysical Research: Solid Earth, 125(11), e2020JB020330. https://doi.org/10.1029/2020JB020330.
Fan, J. F., Shi, J., Wan, X. et al., 2022, Classification evaluation method for Chang 7 oil group of Yanchang formation in Ordos Basin: Journal of Petroleum Exploration and Production Technology, 12(11), 825–834. https://doi.org/10.1007/s13202-021-01338-4.
Feng, S., Niu, X., Liu, F., et al., 2013, Characteristics of Chang7 tight oil reservoir space in Ordos basin and its significance: Journal of Central South University, 44(11), 4574–4580.
Gabàs, A., Macau, A., Benjumea, B., et al., 2016, Joint audio-magnetotelluric and passive seismic imaging of the Cerdanya Basin: Surveys in Geophysics, 37(5), 897–921.
Gassmann, F., 1951, Elasticity of porous media: Vierteljahrsschrder Naturforschenden Gesselschaft, 96, 1–23.
Gomez, C. T., Dvorkin, J., and Vanorio, T., 2010, Laboratory measurements of porosity, permeability, resistivity, and velocity on Fontainebleau sandstones: Geophysics, 75(6), E191–E204.
Gurevich, B., Makarynska, D., de Paula, O. B., et al., 2010, A simple model for squirt-flow dispersion and attenuation in fluid-saturated granular rocks: Geophysics, 75(6), N109–N120.
Guo, M. Q., Fu, L. Y., Ba, J., 2009, Comparison of stress-associated coda attenuation and intrinsic attenuation from ultrasonic measurements: Geophysical Journal International, 178(1), 447–456.
Han, T., 2018, Joint elastic-electrical properties of artificial porous sandstone with aligned fractures: Geophysical Research Letters, 45(7), 3051–3058.
Han, T., Best, A., Sothcott, J., et al. 2011. Joint elastic-electrical properties of reservoir sandstones and their relationships with petrophysical parameters: Geophysical Prospecting, 59(3), 518–535.
Han, T., Wei, Z., and Li, F., 2020, How the effective pore and grain shapes are correlated in Berea sandstones: Implications for joint elastic-electrical modeling: Geophysics, 85(3), MR147–MR154.
Iwamori, H., Ueki, K., Hoshide, T., et al., 2021, Simultaneous analysis of seismic velocity and electrical conductivity in the crust and the uppermost mantle: A forward model and inversion test based on grid search: Journal of Geophysical Research: Solid Earth, 126(9), e2021JB022307.
Ji, X., Wang, H., Ge, Y., et al., 2022, Empirical mode decomposition-refined composite multiscale dispersion entropy analysis and its application to geophysical well log data: Journal of Petroleum Science and Engineering, 208(12), 109495, https://doi.org/10.1016/j.petrol.2021.109495.
Kazatchenko, E., Markov, M., and Mousatov, A., 2004, Joint modeling of acoustic velocities and electrical conductivity from unified microstructure of rocks: Journal of Geophysical Research, 109(B1), B01202, doi:https://doi.org/10.1029/2003JB002443.
Kirichek, A., Chassagne, C., and Ghose, R., 2019, Predicting the dielectric response of saturated sandstones using a 2-electrode measuring system: Frontiers in Physics, 6 (148), doi: https://doi.org/10.3389/fphy.2018.00148.
Leveaux, J., and Poupon, A., 1971, Evaluation of water saturation in shaly formations: The Log Analyst, 12(4), SPWLA–1971–vXIIn4a1.
Li, M., Tang, Y., Bernabé, Y., et al., 2015, Pore connectivity, electrical conductivity, and partial water saturation: network simulations: Journal of Geophysical Research: Solid Earth, 120(6), 4055–4068.
Li, X., Li, C., Li, B., et al., 2020, Response laws of rock electrical property and saturation evaluation method of tight sandstone: Petroleum Exploration and Development, 47(1), 214–224.
Li, Z., 2018, Processing and comprehensive interpretation of geophysical well-logging data: Beijing Geological Publisher (in Chinese).
Liu, Q., Li, P., Jin, Z. et al., 2022, Organic-rich formation and hydrocarbon enrichment of lacustrine shale strata: A case study of Chang 7 Member: Science China Earth Sciences, 65(1), 118–138.
Ma, R., and Ba, J., 2020, Coda and intrinsic attenuations from ultrasonic measurements in tight siltstones: Journal of Geophysical Research, 125(4), e2019JB018825.
Mavko, G., Mukerji, T., and Dvorkin, J., 2009, The rock physics handbook: tools for seismic analysis of porous media: Cambridge University Press.
Monachesi, L., Wollner, U., and Dvorkin, J., 2020, Effective pore fluid bulk modulus at patchy saturation: An analytic study: Journal of Geophysical Research: Solid Earth, 125(1), e2019JB018267, doi. https://doi.org/10.1029/2019JB018267.
Osborn, J. A., 1945, Demagnetizing factors of the general ellipsoid: Physical review, 67(11–12), 351.
Pang, M., Ba, J., Carcione, J., et al., 2019, Estimation of porosity and fluid saturation in carbonates from rock-physics templates based on seismic Q: Geophysics, 84(6), M25–M36.
Pang, M., Ba, J., and Carcione, J. M., 2021a, Seismic identification of tight-oil reservoirs by using 3D rock-physics templates: Journal of Petroleum Science and Engineering, 201(8), 108476.
Pang, M., Ba, J., Carcione, J. M., et al., 2021b, Elastic-electrical rock-physics template for the characterization of tight-oil reservoir rocks: Lithosphere, Special 3, 3341849.
Pang, M., Ba, J., Carcione, J. M. et al., 2022, Acoustic and electrical properties of tight rocks: A comparative study between experiment and theory: Surveys in Geophysics, 43(3), 1761–1791, https://doi.org/10.1007/s10712-022-09730-3.
Pride, S. R., Berryman, J. G., Commer, M. et al., 2017, Changes in geophysical properties caused by fluid injection into porous rocks: Analytical models: Geophysical Prospecting, 65(3), 766–790.
Shi, J., Zou, Y., Cai, Y., et al., 2021, Organic matter enrichment of the Chang 7 member in the Ordos Basin: Insights from chemometrics and element geochemistry: Marine and Petroleum Geology, 135(3–4), 105404.
Solazzi, S.G., Guarracino, L., Rubino, J.G. et al., 2019, Saturation Hysteresis Effects on the Seismic Signatures of Partially Saturated Heterogeneous Porous Rocks: Journal of Geophysical Research: Solid Earth, 124(11), 11316–11335.
Soleymanzadeh, A., Kord, S., and Monjezi, M., 2021, A new technique for determining water saturation based on conventional logs using dynamic electrical rock typing: Journal of Petroleum Science and Engineering, 196(3–4), 107803.
Sun, H., Duan, L., Liu, L., et al., 2019, The influence of micro-fractures on the flow in tight oil reservoirs based on pore-network models: Energies, 12(21), 4104.
Wang, Q., Tao, S., and Guan, P., 2020, Progress in research and exploration & development of shale oil in continental basins in China: Natural Gas Geoscience, 31(3), 417–427.
Wang, S., Tan, M., Wang, X., et al., 2022, Microscopic response mechanism of electrical properties and saturation model establishment in fractured carbonate rocks: Journal of Petroleum Science and Engineering, 208(1), 109429.
Wollner, U., and Dvorkin, J., 2018, Effective bulk modulus of the pore fluid at patchy saturation: Geophysical Prospecting, 66(6), 1372–1383.
Yan, W., Sun, J., and Golsanami, N., et al., 2019, Evaluation of wettabilities and pores in tight oil reservoirs by a new experimental design: Fuel, 252, 272–280.
Yan, W., Sun, J., Zhang, J., et al., 2018, Studies of electrical properties of low-resistivity sandstones based on digital rock technology: Journal of Geophysics and Engineering, 15(1), 153–163.
Yang, H., Niu, X., Xu, L., et al., 2016, Exploration potential of shale oil in Chang7 Member, Upper Triassic Yanchang Formation, Ordos Basin, NW China: Petroleum Exploration and Development, 43(4), 511–520.
Yang, Z., and Zou, C., 2019, Exploring petroleum inside source kitchen: connotation and prospects of source rock oil and gas: Petroleum Exploration and Development, 46(1), 173–184.
Ziarani, A. S., and Aguilera, R., 2012, Pore-throat radius and tortuosity estimation from formation resistivity data for tight-gas sandstone reservoirs: Journal of Applied Geophysics, 83, 65–73.
Zou, C., Zhang, G., Yang, Z., et al., 2013, Geological concepts, characteristics, resource potential and key techniques of unconventional hydrocarbon: on unconventional petroleum geology: Petroleum Exploration and Development, 40(4), 385–399.
Zhang, L., Ba, J., Carcione, J. M., et al., 2019, Estimation of pore microstructure by using the static and dynamic moduli: International Journal of Rock Mechanics and Mining Sciences, 113: 24–30.
Zhang, L., Ba, J., and Carcione, J. M., 2021, Wave propagation in infinituple-porosity media: Journal of Geophysical Research: Solid Earth, 126(4): e2020JB021266.
Zhang, L., Ba, J., Carcione, J. M., et al., 2022, Seismic wave propagation in partially saturated rocks with a fractal distribution of fluid-patch size: Journal of Geophysical Research: Solid Earth, 127(2), e2021JB023809.
Acknowledgments
We thank Dr. Han Xuehui of the China University of Petroleum (East China) for his assistance in the experimental tests. This work is supported by the National Natural Science Foundation of China (Nos. 41974123, 42174161), the Jiangsu Innovation and Entrepreneurship Plan and the Jiangsu Province Science Fund for Distinguished Young Scholars (grant no. BK20200021).
Author information
Authors and Affiliations
Corresponding author
Additional information
Pang Meng-Qiang received his B.S. in Geological Engineering from Tianjin Chengjian University in 2017. He is presently a Ph.D. student in the School of Earth Sciences and Engineering at Hohai University. His research interests are rock physics model, reservoir prediction and acoustic and electrical properties.
Rights and permissions
About this article
Cite this article
Pang, MQ., Ba, J., Wu, CF. et al. Acoustic-electrical properties and rock physics models for shale-oil formations: prediction of reservoir properties of interbedded sandstone and shale layers. Appl. Geophys. 19, 485–502 (2022). https://doi.org/10.1007/s11770-022-0949-z
Received:
Revised:
Published:
Issue Date:
DOI: https://doi.org/10.1007/s11770-022-0949-z