Abstract
With climate change and the ever-drier climate, the issue of wildfires is becoming increasingly prominent, generating growing interest in the study of wildfires. The majority of the ongoing research is focused on surface wildland fuels with particular emphasis on dead and usually dry fuel. These insights are difficult to transpose to live fuels, particularly to crown fires. The flammability properties of dead and dry forest fuels are of little significance for understanding the onset and spread of crown fires. Hence, research regarding the flammability properties of fresh forest vegetation is very sparse. The same observation applies to crown fires, despite the fact that this type of wildfires is devastating, difficult to suppress, and usually having dramatic consequences. The aim of this paper is to determine how moisture dynamics of live crown samples (terminal parts of basal branches) of two coniferous species, Abies alba and Picea abies, influence their flammability properties. Experiments were performed in an adapted mass loss calorimeter with a custom-made sample holder in order to mimic the scenario of initiation of crown fires (surface to crown fire interface). Tests were performed with heat flux values of 50, 60, and 70 kW/m2 and with different moisture levels. At all heat flux values, the results show an increasing trend for the peak heat release rate when moisture content is reduced. A. alba samples reach higher peak release rates in comparison with P. abies samples. At heat fluxes of 50 kW/m2 and 60 kW/m2, fresh A. alba samples take longer to ignite than the P. abies samples. At the heat flux of 70 kW/m2, for the set of analyzed moisture contents, the ignition time interval for the A. alba samples is shorter than for the P. abies samples. The results of the principal component analysis (PCA) show that variables such as time to ignition (TTI), peak heat release rate (PHRR), and mean heat release rate (mean HRR) best describe the ignitability of the analyzed conifer samples.








Similar content being viewed by others
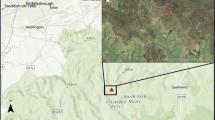
References
Welcome to the Nation’s Logistical Support Center | National Interagency Fire Center. https://www.nifc.gov/ (Accessed 14 May 2022)
Largest wildfires of the decade—BatchGeo Blog. https://blog.batchgeo.com/largest-wildfires/ (Accessed 14 May 2022)
Yao JQ, Zhai HR, Tang XM, Gao XM, Yang XD (2020) Amazon fire monitoring and analysis based on multi-source remote sensing data. IOP Conf Ser. https://doi.org/10.1088/1755-1315/474/4/042025
Lagouvardos K, Kotroni V, Giannaros TM, Dafis S (2019) Meteorological conditions conducive to the rapid spread of the deadly wildfire in Eastern Attica, Greece. Bull Am Meteorol Soc 100(11):2137–2145. https://doi.org/10.1175/BAMS-D-18-0231.1
Ganteaume A, Jappiot M, Lampin C, Guijarro M, Hernando C (2013) Flammability of some ornamental species in wildland-urban interfaces in southeastern France: laboratory assessment at particle level. Environ Manage 52(2):467–480. https://doi.org/10.1007/s00267-013-0067-z
Simpson KJ et al (2016) Determinants of flammability in savanna grass species. J Ecol 104(1):138–148. https://doi.org/10.1111/1365-2745.12503
White RH, Zipperer WC (2010) Testing and classification of individual plants for fire behaviour: plant selection for the wildlandurban interface. Int J Wildl Fire 19(2):213–227. https://doi.org/10.1071/WF07128
Ormeño E et al (2009) The relationship between terpenes and flammability of leaf litter. For Ecol Manage 257(2):471–482. https://doi.org/10.1016/j.foreco.2008.09.019
Della Rocca G, Madrigal J, Marchi E, Michelozzi M, Moya B, Danti R (2017) Relevance of terpenoids on flammability of Mediterranean species: an experimental approach at a low radiant heat flux. IForest 10(5):766–775. https://doi.org/10.3832/ifor2327-010
Morgan Varner J, Kane JM, Kreye JK, Engber E (2015) The flammability of forest and woodland litter: a synthesis. Curr For Rep 1(2):91–99. https://doi.org/10.1007/s40725-015-0012-x
Badmaev N, Bazarov A (2020) Correlation analysis of terrestrial and satellite meteodata in the territory of the Republic of Buryatia (Eastern Siberia, Russian Federation) with forest fire statistics. Agric For Meteorol 297:108245. https://doi.org/10.1016/j.agrformet.2020.108245
Pachauri R, Meyer L (2014) Climate change 2014—synthesis report. Accessed 01 Mar 2021. http://ipcc.ch/pdf/assessment-report/ar5/syr/SYR_AR5_FINAL_full_wcover.pdf
Khabarov N et al (2016) Forest fires and adaptation options in Europe. Reg Environ Chang 16(1):21–30. https://doi.org/10.1007/s10113-014-0621-0
Matthews S (2014) Dead fuel moisture research: 1991–2012. Int J Wildl Fire 23(1):78–92. https://doi.org/10.1071/WF13005
Fernandes PM, Botelho HS, Rego FC, Loureiro C (2009) Empirical modelling of surface fire behaviour in maritime pine stands. Int J Wildl Fire 18(6):698. https://doi.org/10.1071/WF08023
Dimitrakopoulos AP, Papaioannou KK (2001) Flammability assessment of Mediterranean forest fuels. Fire Technol 37(2):143–152. https://doi.org/10.1023/A:1011641601076
Pyne Stephen J, Andrews PL, Laven RD (1996) Introduction to wildland fire. Wiley, New York
Verbesselt J, Fleck S, Coppin P (2002) Estimation of fuel moisture content towards fire risk assessment: a review. In: Viegas DX (ed) Forest fire research & wildland fire safety. Millpress, Rotterdam, pp 1–11
Finney MA, Cohen JD, McAllister SS, Jolly WM (2013) On the need for a theory of wildland fire spread. Int J Wildl Fire 22(1):25–36. https://doi.org/10.1071/WF11117
Xanthopoulos G, Athanasiou M (2020) Crown fire. Encyclopedia of wildfires and wildland-urban interface (WUI) fires. Springer, Cham, pp 1–15
Moreira F, Arianoutsou M, Corona P, las Heras JD (2012) Post-fire management and restoration of Southern European forests, vol 24. Springer, Dordrecht
Rodríguez y Silva F et al (2017) Assessment of crown fire initiation and spread models in Mediterranean conifer forests by using data from field and laboratory experiments. For Syst 26(2):1–14. https://doi.org/10.5424/fs/2017262-10652
Cruz MG (1999) Modeling the initiation and spread of crown fires. Dissertation, University of Montana
Molchanov V (1957) Conditions for the spread of crown fire in pine forest. Lesn Khozydystvo 10:50–63
Van Wagner CE (1977) Conditions for the start and spread of crown fire. Can J For Res 7(1):23–34. https://doi.org/10.1139/X77-004
Van Wagner CE (1993) Prediction of crown fire behavior in two stands of jack pine. Can J For Res 23(3):442–449. https://doi.org/10.1139/x93-062
Keane Robert E (2015) Wildland fuel fundamentals and applications. Springer, Cham
Ferguson SC, Dahale A, Shotorban B, Mahalingam S, Weise DR (2013) The role of moisture on combustion of pyrolysis gases in wildland fires. Combust Sci Technol 185(3):435–453. https://doi.org/10.1080/00102202.2012.726666
Weise DR et al (2018) Fire behavior in chaparral–evaluating flame models with laboratory data. Combust Flame 191:500–512. https://doi.org/10.1016/j.combustflame.2018.02.012
Xanthopoulos G, Wakimoto RH (1993) A time to ignition–temperature–moisture relationship for branches of three western conifers. Can J For Res 23(2):253–258. https://doi.org/10.1139/X93-034
Cruz MG, Alexander ME, Wakimoto RH (2003) Assessing canopy fuel stratum characteristics in crown fire prone fuel types of western North America. Int J Wildl Fire 12(1):39–50. https://doi.org/10.1071/WF02024
Cruz MG, Alexander ME, Wakimoto RH (2004) Modeling the likelihood of crown fire occurrence in conifer forest stands. For Sci 50(5):640–658
Cruz MG, Alexander ME, Wakimoto RH (2003) Assessing the probability of crown fire initiation based on fire danger indices. For Chron 79(5):976–983. https://doi.org/10.5558/tfc79976-5
Cruz MG, Alexander ME, Wakimoto RH (2005) Development and testing of models for predicting crown fire rate of spread in conifer forest stands. Can J For Res 35(7):1626–1639. https://doi.org/10.1139/x05-085
Alexander ME, Cruz MG (2012) Interdependencies between flame length and fireline intensity in predicting crown fire initiation and crown scorch height. Int J Wildl Fire 21(2):95–113. https://doi.org/10.1071/WF11001
Cruz MG, Alexander ME (2010) Assessing crown fire potential in coniferous forests of western North America: a critique of current approaches and recent simulation studies. Int J Wildl Fire 19(4):377–398. https://doi.org/10.1071/WF08132
Cruz MG, Alexander ME, Wakimoto RH (2002) Predicting crown fire behavior to support forest fire management decision-making. For Fire Res Wildl Fire Saf [Online]. http://nofc.cfs.nrcan.gc.ca/bookstore_pdfs/21122.pdf
Cruz M, Alexander M, Wakimoto RH (2003) Definition of a fire behavior model evaluation protocol: a case study application to crown fire behavior models. Fire Fuel Treat Ecol Restor [Online]. http://www.cnr.uidaho.edu/for433/secure/lessons/lesson02/cruzetal_2003_RMRS_proc.pdf
Alexander ME, Cruz MG (2011) Crown fire dynamics in conifer forests. In: Synthesis of knowledge of extreme fire behavior: volume I for fire managers. Department of Agriculture, Forest Service, Pacific Northwest Research Station, Portland, pp 107–144
Cruz MG, Butler BW, Alexander ME, Viegas DX (2006) Development and evaluation of a semi-physical crown fire initiation model. V International conference on forest fire research. https://doi.org/10.1016/j.foreco.2006.08.132
Byram GM (1959) Combustion of forest fuels. Forest fire: control and use. McGraw-Hill Book Company Inc, New York, pp 61–89
Morandini F, Perez-Ramirez Y, Tihay V, Santoni PA, Barboni T (2013) Radiant, convective and heat release characterization of vegetation fire. Int J Therm Sci 70:83–91. https://doi.org/10.1016/j.ijthermalsci.2013.03.011
Sullivan EA, Mcdonald AG (2014) Mathematical model and sensor development for measuring energy transfer from wildland fires. Int J Wildl Fire 23(7):995–1004. https://doi.org/10.1071/WF14016
Scott JH, Reinhardt ED (2005) Stereo photo guide for estimating canopy fuel characteristics in conifer stands. USDA For Serv 145:1–51
Weise DR, Zhou X, Sun L, Mahalingam S (2005) Fire spread in chaparral—‘Go or no-go?’ Int J Wildl Fire 14(1):99–106. https://doi.org/10.1071/WF04049
Cobian-Iñiguez J, Aminfar AH, Saha S, Awayan K, Weise DR, Princevac M (2022) The transition and spread of a chaparral crown fire: insights from laboratory scale wind tunnel experiments. J Combust 2022:1–13. https://doi.org/10.1155/2022/5630594
Weise DR, Koo E, Zhou X, Mahalingam S, Morandini F, Balbi JH (2016) Fire spread in chaparral—a comparison of laboratory data and model predictions in burning live fuels. Int J Wildl Fire 25(9):980–994. https://doi.org/10.1071/WF15177
Sanpakit C, Omodan S, Weise D, Princevac M (2015) Laboratory fire behavior measurements of chaparral crown fire. Univ Calif Riverside Undergrad Res J 9:123–129
Weise DR, Cobian-Iñiguez J, Princevac M (2018) Surface to crown transition. Encyclopedia of wildfires and wildland-urban interface (WUI) fires. Springer, Cham, pp 988–992
Leonelli L, Barboni T, Santoni PA, Quilichini Y, Coppalle A (2017) Characterization of aerosols emissions from the combustion of dead shrub twigs and leaves using a cone calorimeter. Fire Saf J 91:800–810. https://doi.org/10.1016/j.firesaf.2017.03.048
Ganteaume A, Jappiot M, Curt T, Lampin C, Borgniet L (2014) Flammability of litter sampled according to two different methods: comparison of results in laboratory experiments. Int J Wildl Fire 23(8):1061–1075. https://doi.org/10.1071/WF13045
Alam MA et al (2020) Shoot flammability is decoupled from leaf flammability, but controlled by leaf functional traits. J Ecol 108(2):641–653. https://doi.org/10.1111/1365-2745.13289
Simeoni A et al (2012) Flammability studies for wildland and wildland-urban interface fires applied to pine needles and solid polymers. Fire Saf J 54:203–217. https://doi.org/10.1016/j.firesaf.2012.08.005
Fateh T, Richard F, Batiot B, Rogaume T, Luche J, Zaida J (2016) Characterization of the burning behavior and gaseous emissions of pine needles in a cone calorimeter—FTIR apparatus. Fire Saf J 82:91–100. https://doi.org/10.1016/j.firesaf.2016.03.008
Romero B, Ganteaume A (2021) Effect of fire frequency on the flammability of two Mediterranean pines: link with needle terpene content. Plants 10(10):1–23. https://doi.org/10.3390/plants10102164
Jaureguiberry P, Bertone G, Díaz S (2011) Device for the standard measurement of shoot flammability in the field. Austral Ecol 36(7):821–829. https://doi.org/10.1111/j.1442-9993.2010.02222.x
Gigović L, Pourghasemi HR, Drobnjak S, Bai S (2019) Testing a new ensemble model based on SVM and random forest in forest fire susceptibility assessment and its mapping in Serbia’s Tara National Park. Forests 10(5):408. https://doi.org/10.3390/F10050408
Marković T, Radanović D, Nastasijević B, Antić-Mladenović S, Vasić V, Matković A (2019) Yield, quality and safety of yellow gentian roots produced under dry-farming conditions in various single basal fertilization and planting density models. Ind Crops Prod 132:236–244. https://doi.org/10.1016/j.indcrop.2019.02.027
Lode project—Loss data enhancement for DDR & CCA management. https://www.lodeproject.polimi.it/ (Accessed 08 Dec 2020)
Bartoli P, Simeoni A, Biteau H, Torero JL, Santoni PA (2011) Determination of the main parameters influencing forest fuel combustion dynamics. Fire Saf J 46(1–2):27–33. https://doi.org/10.1016/j.firesaf.2010.05.002
Schemel CF, Simeoni A, Biteau H, Rivera JD, Torero JL (2008) A calorimetric study of wildland fuels. Exp Therm Fluid Sci 32(7):1381–1389. https://doi.org/10.1016/j.expthermflusci.2007.11.011
Jervis FX, Rein G (2016) Experimental study on the burning behaviour of Pinus halepensis needles using small-scale fire calorimetry of live, aged and dead samples. Fire Mater 40(3):385–395. https://doi.org/10.1002/fam.2293
Santoni PA, Romagnoli E, Chiaramonti N, Barboni T (2015) Scale effects on the heat release rate, smoke production rate, and species yields for a vegetation bed. J Fire Sci 33(4):290–319. https://doi.org/10.1177/0734904115591176
Simeoni A (2013) Experimental understanding of wildland fires. Fire phenomena and the earth system. Wiley, Hoboken, pp 35–52
Finney MA, Cohen ABJD, Mcallister SS, Matt W (2013) On the need for a theory of wildland fire spread. Int J Wildland Fire. https://doi.org/10.1071/WF11117
ISO (2015) 5660-1. Reaction-to-fire tests – Heat release, smoke production and mass loss rate – Part 1: Heat release rate (cone calorimeter method) and smoke production rate (dynamic measurement)
Babrauskas V (1984) Development of the cone calorimeter—a bench-scale heat release rate apparatus based on oxygen consumption. Fire Mater 8(2):81–95. https://doi.org/10.1002/fam.810080206
McDaniel JK, Alexander HD, Siegert CM, Lashley MA (2020) Shifting tree species composition of upland oak forests alters leaf litter structure, moisture, and flammability. For Ecol Manage 482:118860. https://doi.org/10.1016/j.foreco.2020.118860
Werth PA et al (2011) Synthesis of knowledge of extreme fire behavior: volume I for fire managers. Department of Agriculture, Forest Service, Pacific Northwest Research Station, Portland
Molchanov V (1957) Conditions for the spread of crown fires in pine forests. Lesn Khoziaistvo 10(8):50–53
Keane RE (2019) Canopy fuel. In: Manzello SL (ed) Encyclopedia of wildfires and wildland-urban interface (WUI) fires. Springer, Cham, pp 1–12
Safdari MS, Amini E, Weise DR, Fletcher TH (2019) Comparison of pyrolysis of live wildland fuels heated by radiation vs convection. Fuel 268:117342. https://doi.org/10.1016/j.fuel.2020.117342
Safdari MS (2018) Characterization of pyrolysis products from fast pyrolysis of live and dead vegetation native. Dissertation, Brigham Young University
Safdari MS et al (2018) Characterization of pyrolysis products from fast pyrolysis of live and dead vegetation native to the Southern United States. Fuel 229:151–166. https://doi.org/10.1016/j.fuel.2018.04.166
Butler BW et al (2004) Measurements of radiant emissive power and temperatures in crown fires. Can J For Res 34(8):1577–1587. https://doi.org/10.1139/X04-060
Silvani X, Morandini F, Muzy J-F (2009) Wildfire spread experiments: fluctuations in thermal measurements. Int Commun Heat Mass Transf 36(9):887–892. https://doi.org/10.1016/j.icheatmasstransfer.2009.06.008
McAllister S, Grenfell I, Hadlow A, Jolly WM, Finney M, Cohen J (2012) Piloted ignition of live forest fuels. Fire Saf J 51:133–142. https://doi.org/10.1016/j.firesaf.2012.04.001
Lamorlette A, El Houssami M, Morvan D (2018) An improved non-equilibrium model for the ignition of living fuel. Int J Wildl Fire 27(1):29–41. https://doi.org/10.1071/WF17020
Weise DR, White RH, Beall FC, Etlinger M (2005) Use of the cone calorimeter to detect seasonal differences in selected combustion characteristics of ornamental vegetation. Int J Wildl Fire 14(3):321–338. https://doi.org/10.1071/WF04035
Blank RR, White RH, Ziska LH (2006) Combustion properties of Bromus tectorum L.: influence of ecotype and growth under four CO2 concentrations. Int J Wildl Fire 15(2):227–236. https://doi.org/10.1071/WF05055
Dibble AC, White RH, Lebow PK (2007) Combustion characteristics of north-eastern USA vegetation tested in the cone calorimeter: invasive versus non-invasive plants. Int J Wildl Fire 16(4):426–443. https://doi.org/10.1071/WF05103
Madrigal J, Hernando C, Guijarro M, DÃez C, Marino E, De Castro AJ (2009) Evaluation of forest fuel flammability and combustion properties with an adapted mass loss calorimeter device. J Fire Sci 27(4):323–342. https://doi.org/10.1177/0734904109102030
Ramadhan ML, Zarate S, Carrascal J, Osorio AF, Hidalgo JP (2021) Effect of fuel bed size and moisture on the flammability of Eucalyptus saligna leaves in cone calorimeter testing. Fire Saf J 120:103016. https://doi.org/10.1016/j.firesaf.2020.103016
Romero B, Fernandez C, Lecareux C, Ormeño E, Ganteaume A (2019) How terpene content affects fuel flammability of wildland–urban interface vegetation. Int J Wildl Fire 28(8):614–627. https://doi.org/10.1071/WF18210
Ormeño E et al (2009) The relationship between terpenes and flammability of leaf litter. For Ecol Manage 257(2):471–482. https://doi.org/10.1016/j.foreco.2008.09.019
Pausas JG, Alessio GA, Moreira B, Segarra-Moragues JG (2016) Secondary compounds enhance flammability in a Mediterranean plant. Oecologia 180(1):103–110. https://doi.org/10.1007/s00442-015-3454-8
Zamponi L, Michelozzi M, Capretti P (2007) Terpene response of Picea abies and Abies alba to infection with Heterobasidion s.l. For Pathol 37(4):243–250. https://doi.org/10.1111/j.1439-0329.2007.00493.x
Madrigal J, Guijarro M, Hernando C, Díez C, Marino E (2011) Effective heat of combustion for flaming combustion of mediterranean forest fuels. Fire Technol 47(2):461–474. https://doi.org/10.1007/s10694-010-0165-x
Madrigal J, Hernando C, Guijarro M (2013) A new bench-scale methodology for evaluating the flammability of live forest fuels. J Fire Sci 31(2):131–142. https://doi.org/10.1177/0734904112458244
Etlinger MG, Beall FC (2004) Development of a laboratory protocol for fire performance of landscape plants. Int J Wildl Fire 13(4):479–488. https://doi.org/10.1071/WF04039
El Houssami M et al (2016) Experimental and numerical studies characterizing the burning dynamics of wildland fuels. Combust Flame 168:113–126. https://doi.org/10.1016/j.combustflame.2016.04.004
Yashwanth BL, Shotorban B, Mahalingam S, Lautenberger CW, Weise DR (2016) A numerical investigation of the influence of radiation and moisture content on pyrolysis and ignition of a leaf-like fuel element. Combust Flame 163:301–316. https://doi.org/10.1016/j.combustflame.2015.10.006
Dahale A, Ferguson S, Shotorban B, Mahalingam S (2013) Effects of distribution of bulk density and moisture content on shrub fires. Int J Wildl Fire 22(5):625–641. https://doi.org/10.1071/WF12040
Pickett BM, Isackson C, Wunder R, Fletcher TH, Butler BW, Weise DR (2010) Experimental measurements during combustion of moist individual foliage samples. Int J Wildl Fire 19(2):153–162. https://doi.org/10.1071/WF07121
McAllister S, Weise DR (2017) Effects of season on ignition of live wildland fuels using the forced ignition and flame spread test apparatus. Combust Sci Technol 189(2):231–247. https://doi.org/10.1080/00102202.2016.1206086
Babrauskas V (2006) Effective heat of combustion for flaming combustion of conifers. Can J For Res 36(3):659–663. https://doi.org/10.1139/x05-253
Possell M, Bell TL (2013) The influence of fuel moisture content on the combustion of Eucalyptus foliage. Int J Wildl Fire 22(3):343–352. https://doi.org/10.1071/WF12077
White RH, Weise DR, Frommer S (1996) Preliminary evaluation of the flammability of native and ornamental plants with the cone calorimeter. In: Proceedings of the international conference on fire safety, pp. 256–265
Matt FJ, Dietenberger MA, Weise DR (2020) Summative and ultimate analysis of live leaves from Southern U.S. forest plants for use in fire modeling. Energy Fuels 34(4):4703–4720. https://doi.org/10.1021/acs.energyfuels.9b04107
Jolly WM et al (2012) Relationships between moisture, chemistry, and ignition of Pinus contorta needles during the early stages of mountain pine beetle attack. For Ecol Manage 269:52–59. https://doi.org/10.1016/j.foreco.2011.12.022
Boardman CR, Dietenberger MA, Weise DR (2021) Specific heat capacity of wildland foliar fuels to 434°C. Fuel 292:120396. https://doi.org/10.1016/j.fuel.2021.120396
McAllister S, Weise DR (2017) Effects of season on ignition of live wildland fuels using the forced ignition and flame spread test apparatus. Combust Sci Technol 189(2):231–247. https://doi.org/10.1080/00102202.2016.1206086
Acknowledgements
The manuscript is part of the collaboration within the EU COST Action CA18135 FIRElinks.
Funding
This work was financially supported by the Ministry of Education, Science and Technological Development of the Republic of Serbia, [Grant No. 451-03-68/2022-14/ 200148].
Author information
Authors and Affiliations
Corresponding author
Ethics declarations
Conflict of interests
The authors declare no conflict of interest.
Additional information
Publisher's Note
Springer Nature remains neutral with regard to jurisdictional claims in published maps and institutional affiliations.
Appendix
Appendix
1.1 Scatter Plots for Illustrating the Interrelationship Between Flammability Parameters Across Different Heat Flux and FMC Levels
Rights and permissions
Springer Nature or its licensor (e.g. a society or other partner) holds exclusive rights to this article under a publishing agreement with the author(s) or other rightsholder(s); author self-archiving of the accepted manuscript version of this article is solely governed by the terms of such publishing agreement and applicable law.
About this article
Cite this article
Mišić, N., Protić, M., Cerdà, A. et al. Transition from Surface to Crown Fires: Effects of Moisture Content. Fire Technol 60, 669–700 (2024). https://doi.org/10.1007/s10694-023-01525-1
Received:
Accepted:
Published:
Issue Date:
DOI: https://doi.org/10.1007/s10694-023-01525-1