Chronotropic Incompetence During Exercise Testing as a Marker of Autonomic Dysfunction in Individuals with Early Parkinson’s Disease
Abstract
Background:
An attenuated heart rate response to exercise, termed chronotropic incompetence, has been reported in Parkinson’s disease (PD). Chronotropic incompetence may be a marker of autonomic dysfunction and a cause of exercise intolerance in early stages of PD.
Objective:
To investigate the relationship between chronotropic incompetence, orthostatic blood pressure change (supine – standing), and exercise performance (maximal oxygen consumption, VO2peak) in individuals with early PD within 5 years of diagnosis not on dopaminergic medications.
Methods:
We performed secondary analyses of heart rate and blood pressure data from the Study in Parkinson’s Disease of Exercise (SPARX).
Results:
128 individuals were enrolled into SPARX (63.7±9.3 years; 57.0% male, 0.4 years since diagnosis [median]). 103 individuals were not taking chronotropic medications, of which 90 had a normal maximal heart rate response to exercise testing (155.3±14.0 bpm; PDnon-chrono) and 13 showed evidence of chronotropic incompetence (121.3±11.3 bpm; PDchrono, p < 0.05). PDchrono had decreased VO2peak compared to PDnon-chrono (19.7±4.5 mL/kg/min and 24.3±5.8 mL/kg/min, respectively, p = 0.027). There was a positive correlation between peak heart rate during exercise and the change in systolic blood pressure from supine to standing (r = 0.365, p < 0.001).
Conclusions:
A subgroup of individuals with early PD not on dopaminergic medication had chronotropic incompetence and decreased VO2peak, which may be related to autonomic dysfunction. Evaluation of both heart rate responses to incremental exercise and orthostatic vital signs may serve as biomarkers of early autonomic impairment and guide treatment. Further studies should investigate whether cardiovascular autonomic dysfunction affects the ability to exercise and whether exercise training improves autonomic dysfunction.
INTRODUCTION
Parkinson’s disease (PD) is a neurodegenerative syndrome characterized by the gradual onset of motor symptoms such as bradykinesia, rigidity, tremor, gait difficulties and postural instability, as well as non-motor symptoms such as cognitive impairment, depression, and apathy [1]. In addition, there is evidence of autonomic dysfunction in PD, which worsens as the disease progresses, and negatively impacts activities of daily living and health-related quality of life [1–3]. Manifestations of cardiovascular autonomic dysfunction in PD include orthostatic hypotension (i.e., drop in blood pressure greater than 20 mmHg systolic and/or 10 mmHg diastolic from supine to standing) [4], which may affect every third individual throughout the disease course, and low blood pressure and heart rate (HR) responses to exercise [5], which may limit blood flow to working muscles and thereby facilitate exercise intolerance and negatively impact exercise-induced benefits. Although there are no standardized criteria for chronotropic incompetence, it can be broadly defined as the inability of the heart to increase its rate appropriately for the cardiac output to match the metabolic demands during exertion [6].
There is accumulating evidence that high-intensity endurance exercise may slow the progression of PD [7–10]. However, the prescription of high-intensity endurance exercise may be challenging in individuals with PD with chronotropic incompetence. The prevalence of chronotropic incompetence in PD is unknown, but studies using both treadmill and cycle ergometry exercise testing have consistently shown that some individuals with PD have a reduced maximal HR when compared with age-matched controls [11–15]. Chronotropic incompetence may also contribute to poor exercise capacity, as studies have demonstrated a corresponding reduction in exercise performance among individuals with PD when compared to healthy, age-matched peers [11, 13].
The initial HR increment during exercise is provoked by parasympathetic withdrawal, and subsequent HR increment is secondary to sympathetic activation [16]. Both parasympathetic and sympathetic dysfunction have been reported in PD [17]. The abnormal HR response observed during exercise in some people with PD may be secondary to abnormalities in afferent signals from active muscles to central autonomic regions (e.g., altered exercise pressor reflex) and/or impairment in efferent autonomic pathways to the heart and blood vessels [5].
It is important to identify autonomic dysfunction early in PD if it occurs because it is associated with more rapid disease progression, dementia, falls, hospitalization, and shorter survival time [18]. Early interventions may prevent these complications. Thus, orthostatic hypotension should be actively screened as part of routine standard of care in individuals with PD by measuring the blood pressure and HR supine and after 3 min upon standing [4]. Merola and colleagues demonstrated that asymptomatic orthostatic hypotension was associated with similar impairments in activities of daily living and ambulatory capacity than symptomatic orthostatic hypotension and both groups differed from those without orthostatic hypotension [19], highlighting the need to screen for autonomic dysfunction regardless of symptoms. Abnormal postural changes in blood pressure and blunted HR responses to exercise may identify individuals with PD with cardiovascular autonomic dysfunction early in the disease course.
We performed secondary analyses of HR and blood pressure data collected during the Study in Parkinson’s Disease of Exercise (SPARX, NCT01506479). The SPARX clinical trial is a multicenter, randomized, controlled, single-blinded, Phase II study that was designed to test the feasibility of using high-intensity treadmill exercise to modify symptoms of PD in individuals diagnosed within 5 years not yet taking dopaminergic medication [7, 20]. We sought to investigate the frequency of chronotropic incompetence and its relationship with blood pressure changes with active standing as well as exercise performance during treadmill maximal cardiopulmonary exercise testing (CPET).
METHODS
Standard protocol approvals, registrations, and patient consents
All study activities were approved by the Institutional Review Boards of participating sites (the University of Colorado, the University of Illinois at Chicago, Northwestern University, Rush University Medical Center, and the University of Pittsburgh). Study individuals provided written informed consent before participation in any study procedures. Detailed study procedures have been previously reported[7, 20].
Study individuals
SPARX enrolled de novo individuals with PD within 5 years of diagnosis. Individuals were aged 40–80 years, had a Hoehn and Yahr stage of 1-2, were not expected to begin dopaminergic medication within 6 months of enrollment, and were not participating in moderate-intensity endurance exercise more than three days per week leading up to enrollment into the study. Exclusion criteria included uncontrolled cardiovascular disease or an abnormal stress test result, or failure to meet UK Parkinson’s Disease Society Brain Bank criteria for idiopathic PD [21].
Health history, clinical assessment, and medication usage
Participant clinical and demographic data were obtained prospectively via self-report questionnaires and individuals were assessed by trained clinicians. In addition to the Unified Parkinson’s Disease Rating Scale (UPDRS), we also collected the Movement Disorders Society Unified Parkinson’s Disease Rating Scale (MDS-UPDRS), from which 4 measures related to autonomic dysfunction (i.e., sub-sections 1.10 [urinary dysfunction], 1.11 [constipation], 1.12 [lightheadedness on standing], and 1.13 [fatigue]) were derived for analysis. The use of chronotropic medications was documented during the baseline health history and medical records reviews and was confirmed before the completion of study-related exercise testing or training. Supplementary Table 1 lists the agents with a negative chronotropic effect used by individuals enrolledin SPARX.
Cardiopulmonary exercise testing
Individuals completed a CPET on a treadmill using a modified Gardner Protocol [22]. Before initiation of the CPET, baseline blood pressure was measured after resting supine for 5 min. Then, the participants were asked to sit up then stand up, with brachial blood pressure measured in both positions. The timing of measurement of standing blood pressure was not standardized. The protocol consisted of a constant walking speed. The treadmill speed was increased for a few individuals when it was clear the initial speed was too slow. The treadmill grade was increased by 2% every 2 min until volitional fatigue was reached. Expired gas analyses were sampled to measure oxygen consumption and carbon dioxide production (ParvoMedics TrueOne 2400, Salt Lake City, UT for Chicago and Denver; AEI Technologies, MOXUS, Bastrop, TX for Pittsburgh). HR, blood pressure, and rating of perceived exertion (RPE) were recorded at the end of each stage.
Heart rate monitoring
HR was monitored with an electrocardiogram (ECG) before, during, and after the CPET. HR values were obtained during rest in the supine, seated, and standing positions. HR was recorded during the last 30s of each stage during the CPET. Maximal HR was defined as the highest HR achieved during the CPET. Chronotropic incompetence was defined as a failure to reach 85% of age-predicted maximal heart rate (APMHR) (calculated as 220-age) for our primary analyses which we present in this paper [23]. There are at least two alternative definitions of chronotropic incompetence. These include using a threshold of 80% of APMHR and the Wilkoff method (i.e., chronotropic index) [6], which is used to further support the identification of chronotropic incompetence in the event of suspected poor effort during CPET. Supplemental analyses were performed using both methods. These analyses are presented as Supplemental Material and are very similar to our primary analyses. Potential differences in analyses performed using each method are likely due to reductions in statistical power associated with varying sample sizes per group.
Heart rate response groups
We sought to compare individuals based on their age-adjusted HR response and whether they were or were not taking chronotropic medications. The first group consisted of individuals who were not taking negative chronotropic medications and did not display chronotropic incompetence (PDnon-chrono). The second group consisted of those not taking negative chronotropic medications, but who demonstrated chronotropic incompetence (PDchrono). The third group was defined as those taking medications with a known negative chronotropic effect (PDchronomed) regardless of demonstration of chronotropic incompetence. Data from the PDchronomed group was not used to infer the frequency of chronotropic incompetence because medications with a known negative chronotropic effect (e.g., β-blockers) may result in pharmacologically induced low peak HR during exercise.
Statistical analysis
Participant demographic and clinical characteristics were summarized for each of the 3 groups including mean (SD), median (interquartile range), and frequency (percentage). Continuous variables were compared using a one-way analysis of variance (ANOVA) with a Bonferroni correction (adjusted p-values indicated with the subscript adj), and categorical variables were analyzed with a Chi-squared test. Sub-scores of the MDS-UPDRS were analyzed using a Kruskal-Wallis test. VO2peak data were available for 127 individuals as one data point was identified as an outlier. Correlations between orthostatic blood pressure response (i.e., standing – lying blood pressure) and deviation from APMHR were calculated using Pearson correlation coefficients. These data were analyzed for the entire sample and for each study group (i.e., PDnon-chrono, PDchrono, and PDchronomed).
RESULTS
Demographic and clinical characteristics
One hundred twenty-eight individuals were enrolled in SPARX. Individuals were aged 63.7±9.3 [mean±SD] years, 57% were male, and median disease duration from symptom onset was 1.5 years. The mean Unified Parkinson’s Disease Rating Scale (UPDRS) total score was 23.4±9.0 and 74.2% of the individuals were at Hoehn and Yahr stage 2. There were no differences in demographic or clinical characteristics among the 3 groups (Table 1), except for sex (Chi-square p = 0.029). One hundred three individuals were not taking chronotropic medications. Of these, there were 90 individuals in the PDnon-chrono group (87.4%) and 13 (12.6%) displayed evidence of chronotropic incompetence. Further analysis by the Wilkoff method confirmed that 11 of these 13 individuals in the PDchrono group exhibited chronotropic incompetence. 25 individuals were taking chronotropic medications (19.5% of the total sample). There were 11 men (84.6%) and 2 women in the PDchrono group. In contrast, there were 45 men (50%) and 45 women in the PDnon-chrono group, and 17 men (68.0%) and 8 women in the PDchronomed group.
Table 1
Demographic and clinical characteristics of SPARX individuals
Group 1 | Group 2 | Group 3 | Overall | |
PDnon-chrono | PDchrono | PDchronomed | ||
N | 90 | 13 | 25 | 128 |
Age (s) | 62.7 (9.2) | 67.9 (9.2) | 64.8 (9.4) | 63.7 (9.3) |
Sex, N (%) | ||||
Female | 45 (50.0) | 2 (15.4) | 8 (32.0) | 55 (43.0) |
Male | 45 (50.0) | 11 (84.6) | 17 (68.0) | 73 (57.0) |
BMI (kg·m-2) | 26.8 (4.3) | 25.9 (3.7) | 27.3 (3.9) | 26.8 (4.2) |
Race, N (%) | ||||
Asian | 4 (4.4) | 0 (0.0) | 2 (8.0) | 6 (4.7) |
Black | 3 (3.3) | 0 (0.0) | 2 (8.0) | 5 (3.9) |
White | 81 (90.0) | 13 (100.0) | 21 (84.0) | 115 (89.8) |
Not reported | 2 (2.2) | 0 (0.0) | 0 (0.0) | 2 (1.6) |
Ethnicity, N (%) | ||||
Hispanic | 4 (4.4) | 1 (7.7) | 1 (4.0) | 6 (4.7) |
Not Hispanic | 84 (93.3) | 11 (84.6) | 24 (96.0) | 119 (93.0) |
Not reported | 2 (2.2) | 1 (7.7) | 0 (0.0) | 3 (2.3) |
Time since PD diagnosis, (y), median [IQR] | 0.4 [0.1, 1.1] | 0.3 [0.1, 0.4] | 0.3 [0.1, 1.2] | 0.4 [0.1, 1.0] |
Duration of symptoms (y), median [IQR] | 1.5 [0.9, 2.9] | 1.1 [0.6, 1.8] | 1.6 [1.0, 2.8] | 1.5 [0.9, 2.6] |
Hoehn and Yahr, N (%) | ||||
Stage 1 | 25 (27.8) | 3 (23.1) | 5 (20.0) | 33 (25.8) |
Stage 2 | 65 (72.2) | 10 (76.9) | 20 (80.0) | 95 (74.2) |
MFIS Physical Subscale | 7.9 (6.5) | 8.1 (7.1) | 6.9 (6.2) | 7.7 (6.5) |
UPDRS | ||||
UPDRS Total Score | 23.1 (9.2) | 25.9 (7.0) | 23.1 (8.9) | 23.4 (9.0) |
UPDRS Part 1 Score | 0.8 (1.1) | 1.4 (1.8) | 0.8 (1.0) | 0.8 (1.2) |
UPDRS Part 2 Score | 6.2 (3.6) | 5.6 (3.3) | 5.1 (3.3) | 5.9 (3.5) |
UPDRS Part 3 Score | 16.2 (7.0) | 18.9 (5.3) | 17.2 (7.4) | 16.7 (7.0) |
BMI, body mass index; PD, Parkinson’s disease; IQR, inter-quartile range; MFIS, Modified Fatigue Impact Scale; UPDRS, Unified Parkinson’s Disease Rating Scale. Data are mean (SD). For sex, p = 0.029 (Chi-squared test).
Autonomic function and orthostatic vital signs
Sub-scores of the MDS-UPDRS were not different among groups. Lying, seated, or standing blood pressures were also not different between the 3 groups. There was a significant difference in the orthostatic change in systolic blood pressure (standing – lying) between the 3 groups (F = 3.949, p = 0.022, Table 2). Post hoc testing showed a greater reduction in systolic blood pressure with standing in the PDchrono group (–14.2±19.1 mmHg) compared to the PDnon-chrono group (–2.9±12.6 mmHg, p = 0.018adj, Table 2). There were differences in resting HR in the lying, seated, and standing positions between the 3 groups (F[2,116] = 10.869, p < 0.001; F[2,124] = 9.282, p < 0.001; F[2,123] = 10.964, p < 0.001 for lying HR, sitting HR, and standing HR, respectively). Post hoc testing showed an increased lying HR in PDnon-chrono (73.0±9.5 bpm) when compared to PDchrono (61.5±7.4 bpm, p < 0.001adj) and PDchronomed (66.1±10.2 bpm, p = 0.006adj), an increased seated HR in PDnon-chrono (77.7±14.3 bpm) when compared to PDchrono (64.7±10.3 bpm, p = 0.004adj) and PDchronomed (67.8±10.6 bpm, p = 0.004adj), and an increased standing HR in PDnon-chrono (84.3±14.9 bpm) when compared to PDchrono (71.4±9.9 bpm, p = 0.010adj) and PDchronomed (71.6±11.8 bpm, p < 0.001adj).
Table 2
Clinical assessment of autonomic function, orthostatic vital signs, and heart rate response during CPET of SPARX individuals
Group 1 | Group 2 | Group 3 | Overall | |
PDnon-chrono | PDchrono | PDchronomed | ||
N | 90 | 13 | 25 | 128 |
MDS-UPDRS, mean (SD), median (IQR) | ||||
Part 1.10 Score (Urinary Dysfunction)1 | 0.6 (0.8), 0 (0, 1) | 0.2 (0.4), 0 (0, 0.50) | 0.6 (0.7), 0 (0, 1) | 0.5 (0.7), 0 (0, 1) |
Part 1.11 Score (Constipation)1 | 0.4 (0.7), 0 (0, 1) | 0.5 (0.5), 0 (0, 1) | 0.3 (0.6), 0 (0, 0.50) | 0.4 (0.7), 0 (0, 1) |
Part 1.12 Score (Lightheadedness on Standing)1 | 0.3 (0.5), 0, (0, 0.25) | 0.6 (1.0), 0, (0, 1) | 0.2 (0.4), 0 (0, 0) | 0.3 (0.5), 0 (0, 0) |
Part 1.13 Score (Fatigue)1 | 0.7 (0.6), 1 (0, 1) | 0.3 (0.5), 0 (0, 1) | 0.7 (0.7), 1 (0, 1) | 0.6 (0.6), 1 (0, 1) |
Lying SBP, mmHga | 127.4 (15.3) | 129.9 (17.5) | 130.0 (15.7) | 128.2 (15.6) |
Lying DBP, mmHga | 78.4 (10.3) | 77.1 (10.4) | 77.0 (8.8) | 78.0 (10.0) |
Lying HR, bpmb | 73.0 (9.5) | 61.5 (7.4) | 66.1 (10.2) | 70.4 (10.2) |
Seated SBP, mmHgc | 124.6 (15.8) | 119.2 (16.3) | 125.4 (18.4) | 124.2 (16.4) |
Seated DBP, mmHgc | 77.4 (10.4) | 73.7 (10.9) | 76.6 (11.8) | 76.9 (10.7) |
Seated HR, bpmc | 77.7 (14.3) | 64.7 (10.3) | 67.8 (10.6) | 74.4 (14.1) |
Standing SBP, mmHgc | 125.5 (15.7) | 115.7 (17.2) | 124.8 (15.7) | 124.4 (16.0) |
Standing DBP, mmHgc | 78.7 (10.5) | 73.5 (11.3) | 76.7 (12.3) | 77.8 (11.0) |
Standing HR, bpmd | 84.3 (14.9) | 71.4 (9.9) | 71.6 (11.8) | 80.5 (15.0) |
Δ SBP (Standing-Lying), mmHge | –2.9 (12.6) | –14.2 (19.1) | –5.2 (13.8) | –4.5 (13.9) |
Δ DBP (Standing-Lying), mmHge | 0.0 (5.7) | –3.5 (8.9) | –0.2 (8.6) | –0.4 (6.7) |
Δ HR (Standing-Lying), bpmf | 10.6 (8.6) | 8.3 (5.6) | 5.8 (6.9) | 9.4 (8.2) |
Deviation from APMHR (Maximal HR-APMHR) | –2.0 (11.2) | –30.8 (7.7) | –17.8 (18.8) | –8.0 (16.1) |
Δ HR / Δ SBP (Standing-Lying)g | 0.33 (4.28) | 0.31 (1.15) | 0.74 (2.80) | 0.41 (3.81) |
1Kruskal Wallis test used; aLying BP data included n = 126; bLying HR data included n = 119; cSeated BP, Seated HR, and Standing BP data included n = 127; dStanding HR data included n = 126; eΔ SBP and Δ DBP data included n = 125; fΔ HR data included n = 117; gΔHR/ΔSBP data included n = 105. MDS-UPDRS, Movement Disorders Society Unified Parkinson’s Disease Rating Scale; SBP, systolic blood pressure; mmHg, millimeters of mercury; DBP, diastolic blood pressure; APMHR, age-predicted maximal heart rate. Data are mean (SD). For lying HR, p < 0.001 for PDnon-chrono vs. PDchrono, and p = 0.006 for PDnon-chrono vs. PDchronomed; For seated HR, p = 0.004 for PDnon-chrono vs. PDchrono, and p = 0.004 for PDnon-chrono vs. PDchronomed; For standing HR, p = 0.010 for PDnon-chrono vs. PDchrono, and p < 0.001 for PDnon-chrono vs. PDchronomed; For ΔSBP, p = 0.018 for PDnon-chrono vs. PDchrono; For ΔHR, p = 0.034 for PDnon-chrono vs. PDchronomed; For deviation from APMHR, p < 0.001 for PDnon-chrono vs. PDchrono, p < 0.001 for PDnon-chrono vs. PDchronomed, and p = 0.011 for PDchrono vs. PDchronomed.
Cardiopulmonary exercise testing characteristics
All three groups followed the general trend of a reduction in maximal HR with age. There was considerable variability in maximal HR across ages 40–80 years in all three groups. Some individuals had a HR well above the APMHR whereas others had a HR markedly lower than expected for age (Fig. 1). There was a difference in the maximal HR among the 3 groups (F = 34.310, p < 0.001, Fig. 2). Post hoc testing showed that the mean maximal HR during CPET in the PDchrono group (121.3±11.3 bpm [mean±SD]) was lower than PD individuals in both the PDnon-chrono (155.3±14.0 bpm, p < 0.001adj) and PDchronomed (137.4±22.1 bpm, p = 0.010adj) groups (Fig. 2). Individuals in the PDchrono group also achieved a maximal HR that was markedly below APMHR (152 bpm, based on group mean age). There was a difference in maximal HR during CPET among the 3 groups according to the Wilkoff method (F[2,125] = 28.105, p < 0.001). Post hoc testing showed that mean maximal HR during CPET in the PDchronoWilk group (121.1±12.4 bpm) was lower than those in both the PDnon-chronoWilk (154.6±14.6 bpm, p < 0.001adj) and PDchronomed (137.4±22.1 bpm, p = 0.019adj) groups.
Fig. 1
Association between age and maximal heart rate during CPET in SPARX by chronotropic group. PDnon-chrono (n = 90), PDchrono (n = 13), and PDchronomed (n = 25).
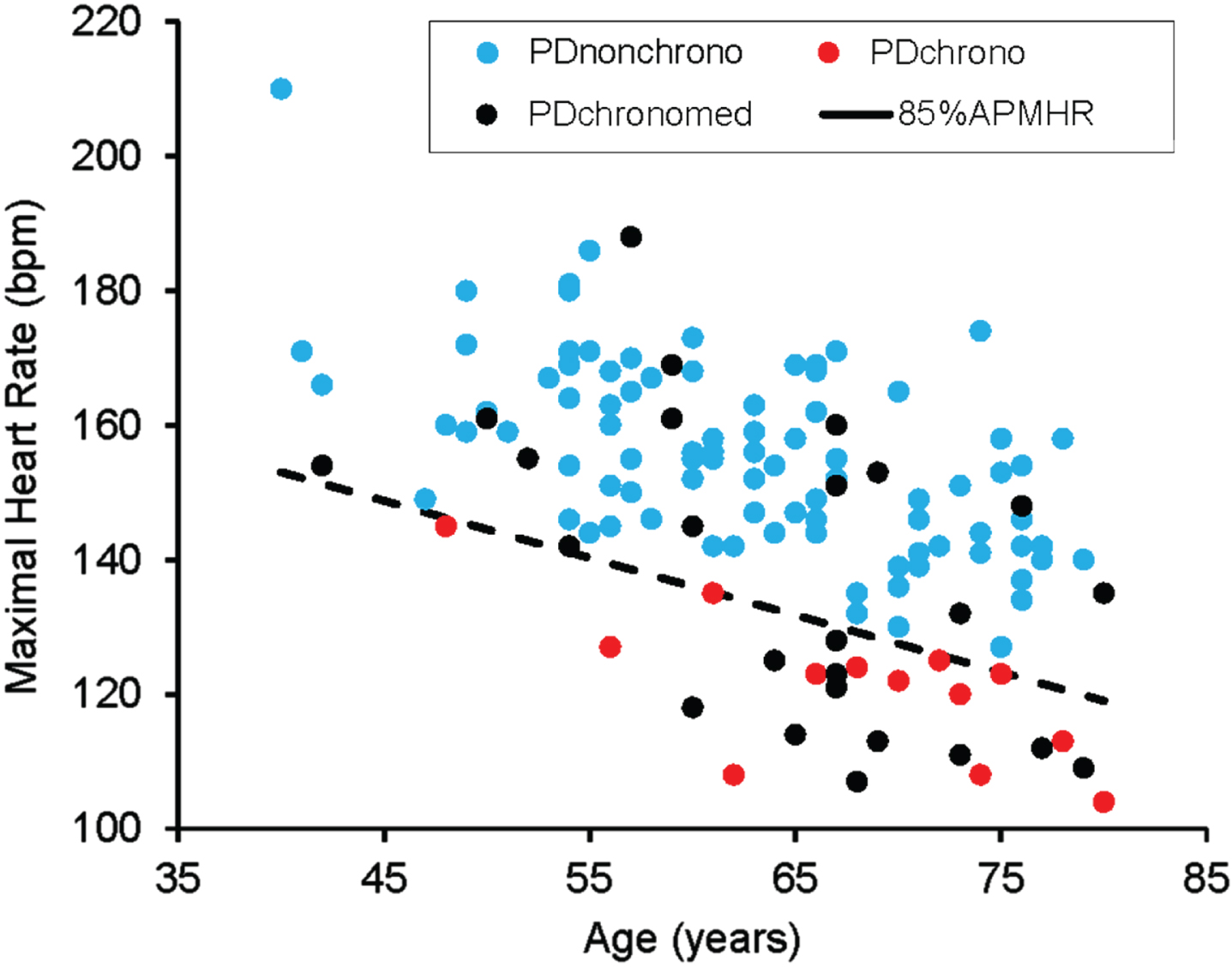
Fig. 2
Maximal heart rate in each study group. Data are median, interquartile range, and 95% confidence interval. PDnon-chrono (n = 90), PDchrono (n = 13), and PDchronomed (n = 25). p < 0.001 for PDnon-chrono vs. PDchrono, p < 0.001 for PDnon-chrono vs. PDchronomed, p = 0.010 for PDchrono vs. PDchronomed.
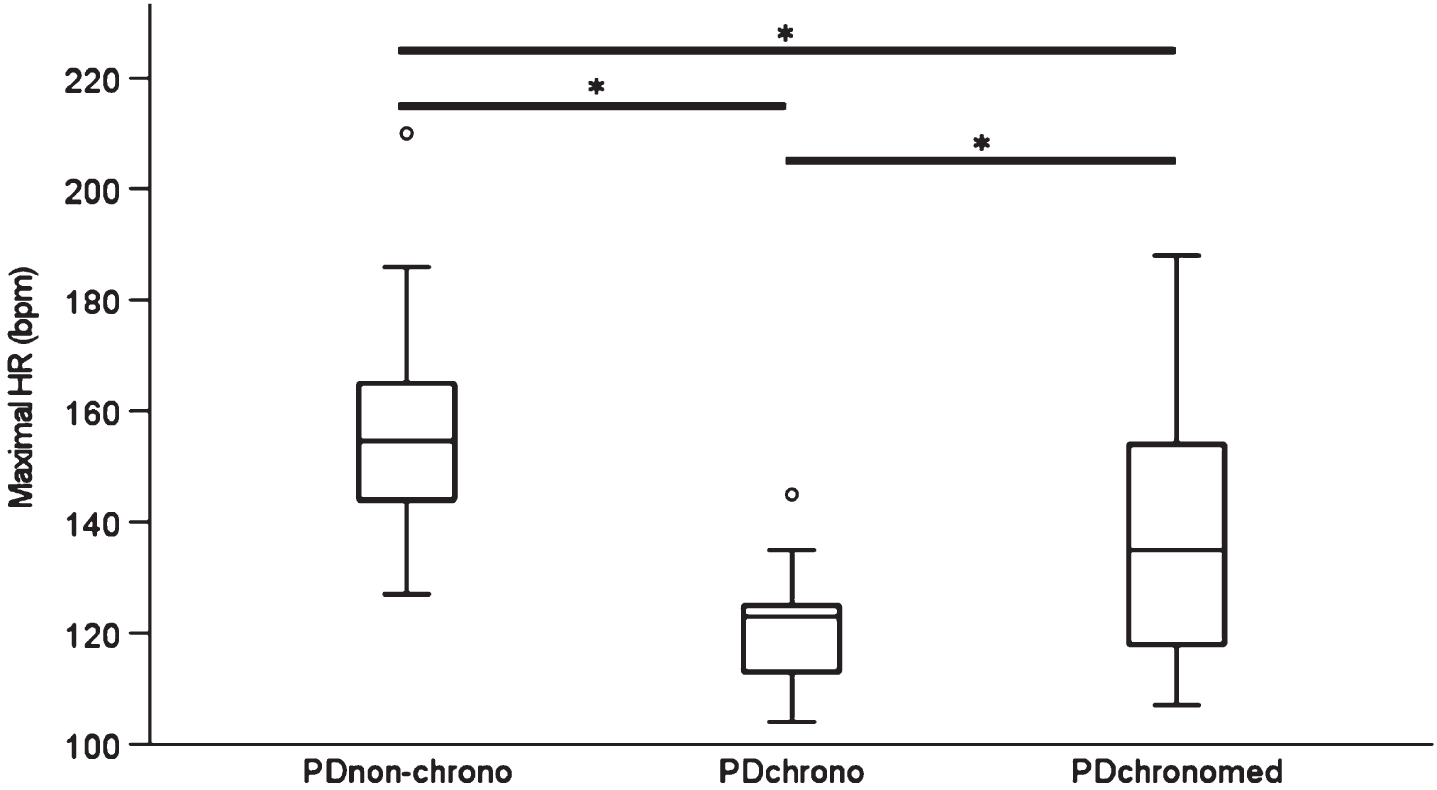
Individual values for the orthostatic change in systolic blood pressure were weakly and positively correlated with the maximal HR response during CPET relative to the APMHR (maximum HR – APMHR) (r = 0.365, p < 0.001). Additional subgroup analyses showed that deviation from APMHR was associated with systolic blood pressure orthostatic change in both the PDchrono (r = 0.581, p = 0.037) and PDchronomed (r = 0.462, p = 0.020) groups, but not in the PDnon-chrono group (r = 0.195, p = 0.070) (Fig. 3).
Fig. 3
Association between systolic orthostatic blood pressure response (i.e., standing-lying SBP) and deviation from APMHR in study participants. PDnon-chrono (n = 87), PDchrono (n = 13), and PDchronomed (n = 25).
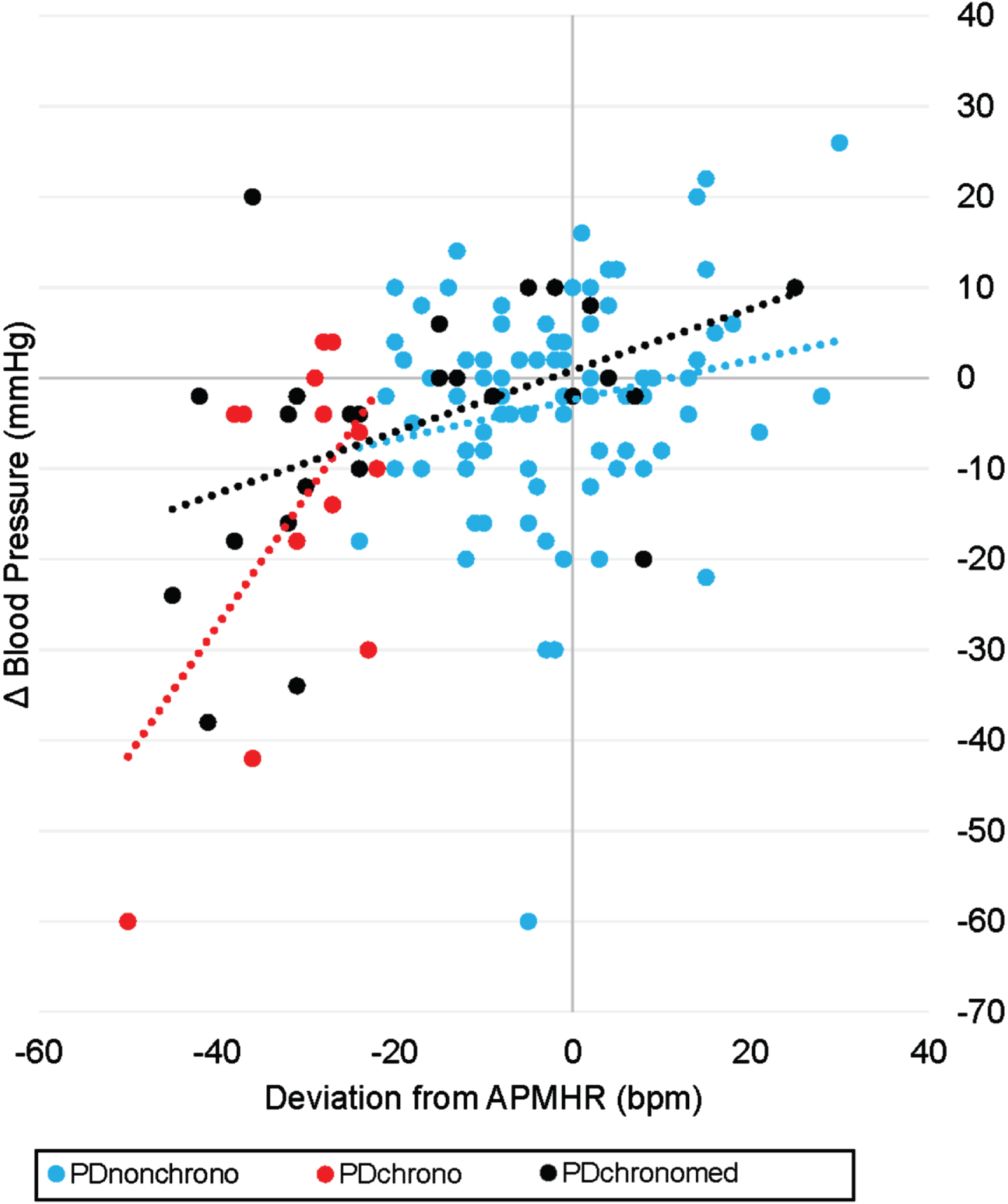
All three groups walked at a similar treadmill speed (F[2,125] = 0.818, p = 0.444), but there were differences among the groups in treadmill grade (F[2,125] = 5.591, p = 0.005) and exercise test duration (F[2,125] = 6.259, p = 0.003). Post hoc testing showed that the mean treadmill grade for the PDchrono group was 7.9±2.8%, which was lower than those in the PDnon-chrono (11.2±3.5%, p = 0.003adj) and PDchronomed (10.7±3.2%, p = 0.041adj) groups. Post hoc testing also revealed that the mean test duration for the PDchrono group was 9.3±3.1 min, which was shorter than the PDnon-chrono (12.5±3.1 min, p = 0.002adj) and PDchronomed (11.9±3.0 min, p = 0.047adj) groups. Full details of CPET are found in Table 3.
Table 3
Cardiopulmonary exercise testing characteristics in SPARX individuals
Group 1 | Group 2 | Group 3 | Overall | |
PDnon-chrono | PDchrono | PDchronomed | ||
N | 90 | 13 | 25 | 128 |
Resting Heart Rate, bpm | 75.1 (10.0) | 62.9 (11.4) | 67.2 (11.3) | 72.3 (11.2) |
Peak Treadmill Speed, mph | 2.9 (0.6) | 2.9 (0.5) | 3.1 (0.7) | 3.0 (0.6) |
Peak Treadmill Grade, % | 11.2 (3.5) | 7.9 (2.8) | 10.7 (3.2) | 10.8 (3.5) |
Exercise Duration, min | 12.5 (3.1) | 9.3 (3.1) | 11.9 (3.0) | 12.0 (3.2) |
Maximal HR, bpm | 155.3 (14.0) | 121.3 (11.3) | 137.4 (22.1) | 148.3 (19.3) |
VO2peak, mL/kg/mina | 24.3 (5.8) | 19.7 (4.5) | 23.8 (6.7) | 23.8 (6.0) |
Peak RER | 1.06 (0.07) | 1.03 (0.07) | 1.06 (0.06) | 1.06 (0.07) |
RPE (n with maximal effort, %)b | 51 (56.7) | 3 (23.1) | 11 (44.0) | 65 (50.8) |
aVO2peak data included n = 127 as 1 data point was determined to be an outlier; bMaximal effort defined by an RPE≥18/20, or an RPE > 8/10. bpm, beats per minute; HR, heart rate; mph, miles per hour; min, minutes; mL/kg/min, milliliters of oxygen per kilogram of body weight per minute; RER, respiratory exchange ratio; RPE, rating of perceived exertion. Data are mean (SD).
There were also differences among the groups in cardiorespiratory fitness (F[2,124] = 3.526, p = 0.032). Post hoc testing showed that VO2peak for the PDchrono group was 19.7±4.5 mL/kg/min which was lower than the PDnon-chrono group (24.3±5.8 mL/kg/min, p = 0.027adj) (Fig. 4A). CPET effort as quantified by the peak respiratory exchange ratio did not differ among groups (F[2,125] = 0.809, p = 0.448) (Fig. 4B). Further, there was no difference in percentage of tests that achieved maximal effort according to rating of perceived exertion (RPE) among groups (i.e., RPE > 8/10 or≥18/20, p = 0.058). The Wilkoff method was used as an additional level of analysis for those in the PDchrono group who had a respiratory exchange ratio < 1.05 and for whom submaximal exercise testing data were available. Supplementary Figure 1 illustrates how the 11 individuals in the PDchronoWilk group were identified [6].
Fig. 4
A) Cardiorespiratory fitness as measured by VO2peak, p = 0.027 for PDnon-chrono vs. PDchrono (PDnon-chrono (n = 89), PDchrono (n = 13), and PDchronomed (n = 25)). B) peak respiratory exchange ratio, p = 0.448 (PDnon-chrono (n = 90), PDchrono (n = 13), and PDchronomed (n = 25). Data are median, interquartile range, and 95% confidence interval.
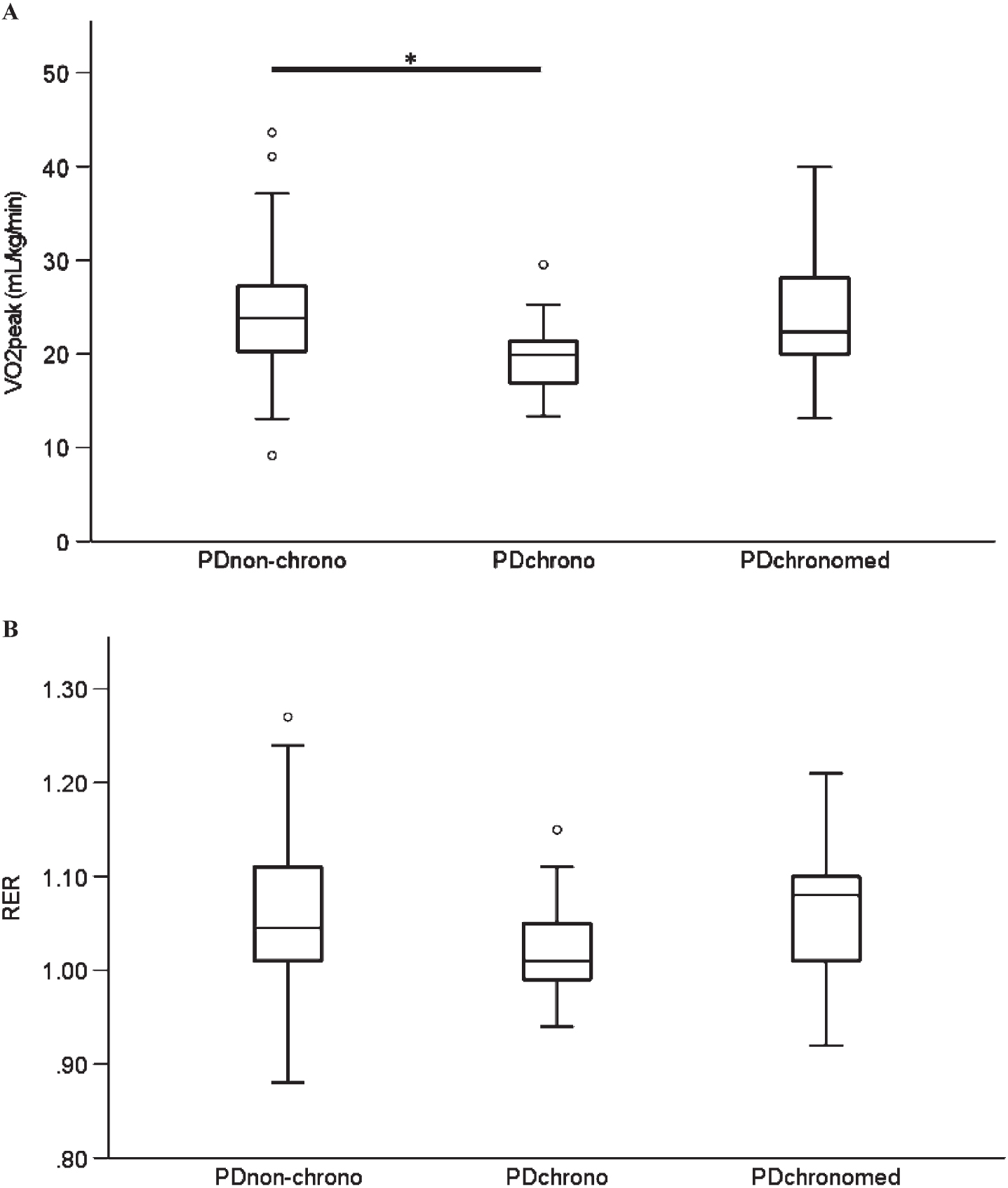
DISCUSSION
The results of this study suggest that a subset of individuals with early PD aged 40–80 years and not on dopaminergic medication has evidence of decreased maximal HR (chronotropic incompetence) during maximal CPET on a treadmill (Fig. 1). Individuals with PD who exhibit an attenuated maximal HR response experienced a greater reduction in systolic BP in response to an orthostatic challenge (i.e., moving from supine to standing) compared to those without chronotropic incompetence (Fig. 3). Chronotropic incompetence in these individuals may reflect early autonomic dysfunction and may be responsible for lower exercise performance.
Decreased maximal HR has been reported in previous studies of individuals with PD compared to age-matched healthy individuals [11–15]. The present study expands on these findings by presenting the presence of chronotropic incompetence in individuals with early PD not on dopaminergic medications. In contrast, analysis of studies that included individuals in a similar age range to the SPARX study revealed that chronotropic incompetence occurred infrequently in healthy individuals [24–26]. A challenge in studies of chronotropic incompetence has been a lack of consistent methodology for determining chronotropic incompetence. The findings of the present study were consistent regardless of the method used to define chronotropic incompetence [6]. In the clinical setting, using an established percentage of either 85% or 80% of the age-predicated maximal HR may identify individuals with PD with cardiovascular autonomic dysfunction.
The relationship between chronotropic incompetence and orthostatic blood pressure changes suggests autonomic dysfunction in a subset of individuals with early PD. Our data support that those with chronotropic incompetence experience a greater drop in systolic BP when moving from supine to standing (Fig. 3). However, this finding should be interpreted as preliminary and needs to be confirmed in a larger cohort in which measurements of vital signs are standardized (e.g., blood pressure measured after 3 min of standing). Initial orthostatic hypotension detected within the first minute of standing is common and often reflects a physiological response to standing caused by a sudden increase of gravitational force [27]. On the other hand, a sustained excessive drop in blood pressure at 3 min (“classic” orthostatic hypotension) or after 5 min of standing (delayed orthostatic hypotension) reflects inadequate compensatory neurocirculatory responses to postural change due to autonomic dysfunction [28].
Orthostatic hypotension in PD is secondary to impaired sympathetic nerve activity with insufficient norepinephrine release from sympathetic postganglionic neurons upon standing up [4]. Impaired sympathetic activity during exercise may also be responsible for chronotropic incompetence; however, the underlying pathophysiologic mechanisms that contribute to the blunted HR responses to exercise in PD are still not fully understood. Sabino-Carvalho and colleagues demonstrated an attenuation of the metabolic component of the exercise pressor reflex in PD compared to controls, while the blood pressure and total peripheral resistance responses to the cold pressor test were similar between PD and controls [29]. These findings suggest impaired afferent signaling during isometric exercise in PD [29]. However, the results of the study by Sabino-Carvalho should be interpreted as preliminary due to the low sample size (11 individuals with PD), the use of isometric handgrip exercise which does not stimulate HR to the same degree as endurance exercise, the lack of control for dopaminergic medications, and the absence of recording of sympathetic nerve activity with microneurography [29]. Further studies should investigate the neural mechanisms responsible for blunted cardiovascular responses during endurance exercise in PD. Besides an abnormal metaboreflex-mediated pressor response, other possible contributors include impairment of the central command, vascular sympathetic and cardiovagal baroreflex dysfunction, cardiac sympathetic denervation, blunted sympathetic norepinephrine release, lower alpha-adrenergic responsiveness, and impairment of peripheral and central chemoreflexes [30–32]. Impaired sympathetic activation can be identified in individuals with orthostatic hypotension by dividing the change in HR by the fall in systolic blood pressure at the 3-min mark from supine to standing [33]. In the present study, the ΔHR/ΔSBP ratio was not different between the PDchrono and PDnon-chrono groups, but most individuals with PD in the SPARX study did not have orthostatic hypotension.
Multiple studies have shown abnormal HR and electrophysiological changes in the prodromal phase of PD [34, 35]. Palma and colleagues demonstrated that the maximal HR response to exercise testing is decreased when compared to age-matched controls in the premotor phase of PD, suggesting the presence of autonomic dysfunction as early as 4.3 years before PD diagnosis [12]. There is evidence that the prevalence of autonomic dysfunction in PD increases as the disease progresses [36], and that this dysfunction may manifest as HR- and blood pressure-related differences when compared to those with normal hemodynamic responses to exercise [11, 13, 15, 37]. Baschieri and colleagues performed cardiovascular reflex tests in 105 people with PD within 3 years from motor onset and reported that 7.8% of individuals had neurogenic orthostatic hypotension after 16 months of follow-up with progressive worsening of sympathetic function [38]. The present study confirms autonomic impairment in a subset of people with early PD, which manifests as impaired cardiovascular responses during orthostatic stress and exercise (Figs. 3 and 4A).
Assessment of autonomic symptoms with questionnaires (e.g., MDS-UPDRS part 1) may not be sensitive to detecting early manifestation of cardiovascular autonomic impairment in PD. On the other hand, the measurement of orthostatic vital signs should be part of the routine clinical evaluation in PD. Importantly, most individuals with chronotropic incompetence in the present study did not have orthostatic hypotension (i.e., drop in blood pressure greater than 20 mmHg systolic and/or 10 mmHg diastolic from supine to standing). Further studies should investigate if lower cutoffs for orthostatic blood pressure changes would identify individuals with early PD with chronotropic incompetence. Early identification and treatment of autonomic dysfunction may prevent future complications including falls, dementia, and hospitalization [18, 39, 40]. The presence of cardiovascular autonomic impairment early in the disease process may also support the hypothesis that some individuals with PD have a more widespread involvement across peripheral and central nervous system locations [41]. Therefore, the identification of autonomic dysfunction by evaluating both HR response to incremental exercise and orthostatic blood pressure changes in early PD may have important prognostic value and assist in tailoring exercise prescriptions and treatment options. Indeed, dopamine agonist therapy can cause or exacerbate orthostatic hypotension, especially during initiation or up-titration [42], whereas this issue has remained controversial with levodopa (the most commonly prescribed medication in PD) [43]. Further studies need to investigate the consequence of cardiovascular autonomic impairment in PD in terms of disease prognosis andmanagement.
Chronotropic incompetence is problematic when clinicians develop personalized endurance exercise prescriptions in PD based on APMHR. Individuals with PD with reduced maximal HR may have difficulty following a prescription based on APMHR, as the calculated value may be an unrealistic and unobtainable HR. One alternative exercise prescription method would be using the percentage of the measured maximal HR. Some individuals with PD may benefit from an objective clinical assessment of maximal HR via CPET to guide exercise prescriptions. Additional research is needed to better identify PD individuals who are more likely to exhibit chronotropic incompetence, so clinicians can perform a CPET and provide appropriate endurance exercise prescriptions.
In people with heart failure, chronotropic incompetence is an important cause of exercise intolerance and an independent predictor of major adverse cardiovascular events and mortality [6]. In the present study, those with chronotropic incompetence had lower cardiorespiratory fitness (Fig. 4A). This reduction in VO2peak of 4.6 mL/kg/min is greater than the 2.2 mL/kg/min reduction that has been reported in heart failure patients who demonstrate chronotropic incompetence [25]. This decrease in fitness may have important clinical implications and contribute to decreased work output for a given HR in some individuals with PD [44, 45]. Individuals with PD have been shown to have reduced VO2peak [13] and exercise duration [11] during maximal exercise testing when compared to those without PD. The present study shows similar reductions in exercise capacity alongside reductions in maximal HR among a subset of adults recently diagnosed with PD and not yet on medications. Further research is necessary to investigate the impact of cardiovascular autonomic dysfunction on exercise tolerance in PD. It is also unknown if people with PD with autonomic dysfunction experience the same benefits from endurance exercise training as their counterparts without autonomic dysfunction. Cardiovascular autonomic dysfunction may improve with exercise training, as shown by Keteyian and colleagues who demonstrated that peak HR increased following exercise training with partial reversal of chronotropic incompetence among individuals with stable heart failure [46]. Whether the same is true in PD is unknown. SPARX3, a multi-center Phase III trial in exercise training in PD and the follow-up study to SPARX, is positioned to answer both important questions and provide additional insight into potential improvement in autonomic dysfunction in these patients.
Approximately 20% of individuals with early PD in SPARX were prescribed medications with a known negative chronotropic effect (Supplementary Table 1), which is comparable to the 22% of adults taking beta-blockers in the non-PD population [47]. The type, dosage, and duration of beta-blocker therapy may impact a participant’s perceived effort for a given bout of endurance exercise [48]. Despite being on beta-blocker medications, 14 individuals (56%) were able to achieve≥85% of APMHR. Of these 14 individuals, 9 (64%) achieved an RER≥1.05. Like other studies, albeit in different patient populations [49, 50], this finding suggests that beta-blockers may not cause chronotropic incompetence. The pathogenesis of chronotropic incompetence may involve mechanisms other than cardioinhibition from β-1 receptor downregulation and desensitization including impairment of the central command, arterial baroreflex, muscle metaboreflex, or peripheral vascular response to exercise. The current findings highlight the need for further studies to investigate the pathogenesis of chronotropic incompetence. Additional research is also required to determine if responses to exercise differ based on chronotropic medication status in PD, and further how to best prescribe exercise intensity in these individuals. SPARX3 will address this important question.
While this study included a relatively large sample size of recently diagnosed individuals with PD who were not taking dopaminergic medications, four methodological considerations are important: 1) lack of comprehensive cardiac testing, 2) determining a true maximal effort, 3) the possibility of impaired motor coordination, and 4) disproportionate sex distributions in the PDchrono group. We will address these methodological considerations in turn.
First, there exists the possibility of cardiac causes of chronotropic incompetence (i.e., heart failure); however, poorly controlled or unstable cardiovascular disease and uncontrolled hypertension were exclusion criteria, and all individuals were evaluated by a physician. In addition, the CPETs were all overseen by a cardiologist, or a physician trained in ECG interpretation.
Second, it is important to recognize that some study individuals may have provided a submaximal effort during CPET as evidenced by the distribution of respiratory exchange ratio (RER) values in each group. As such, it is possible that not every participant identified as having a decreased maximal HR during CPET had chronotropic incompetence. The mean difference in RER between the PDnon-chrono and PDchrono groups was 0.03, and there were no significant differences among groups (p = 0.448). This observation is comparable to data reported in heart failure and may not be clinically meaningful [51], supporting that poor effort during CPET does not explain decreased maximal HR in the PDchrono group. Importantly, the RER and CI relationship is not well-defined in the literature. It is important that future studies monitor CPET administration and performance and establish criteria for maximal efforts. A commonly used criterion is an RER value of 1.05 [51], which may help increase confidence in identifying chronotropic incompetence. In the current study, we present supplemental analyses using alternative criteria for chronotropic incompetence, specifically the Wilkoff method (chronotropic index) (Supplementary Tables 2–5). These analyses confirm that, even with the application of more conservative approaches to the identification of chronotropic incompetence, a subset of recently diagnosed PD patients still shows attenuated HR responses to maximal exercise.
The Wilkoff method relies upon the analysis of submaximal exercise data from each stage of a CPET and provides an approach to further analyze the relationship between HR response and metabolic effort during exercise instances in which low RER may be reported. The application of this method to our SPARX data resulted in 11 PD patients being categorized as having chronotropic incompetence compared to 13 patients in our primary analysis (with criteria for chronotropic incompetence as being a failure to achieve 85% of APMHR), supporting our approach with supplemental analysis of metabolic data during exercise. Future studies should establish clear and consistent maximal criteria for CPET, including HR-, RER-, and VO2 plateau-guided metrics to evaluate the effort provided during CPET. The maximal CPET was performed in three different centers, which may have introduced variability in data collection and testing protocol; however, cardiopulmonary exercise testing was performed according to guidelines and supervised by trained investigators [52]. Importantly, out of the 13 individuals in the PDchrono group, none reported any dizziness, and no instances of hypotension were documented. Further, no individuals in this group experienced ECG changes. The most common patient-reported reason for stopping the CPET was “fatigue.”
Third, there is always the possibility that when studying people with PD, impaired motor coordination could limit a person’s ability to achieve a maximal CPET. We do not think this is likely in the individuals we studied as all our individuals were Hoehn and Yahr Stages 1-2 (25.8% in Stage 1 in the total study sample), meaning that their balance was not seriously impacted. Several studies have been conducted using both treadmills and cycle ergometers in which lower maximal HR responses during exercise testing have been identified in PD compared to healthy controls [11–15]. Since the reductions in maximal HR in PD have been shown using both exercise testing modalities, impaired motor coordination related to exercise testing mode would appear an unlikely explanation.
Lastly, we do not know how sex-based differences may affect the results of the study. Our proportion of males to females in the overall study sample is consistent with the literature on the prevalence of PD. While it is well-established that PD prevalence is higher in men compared to women [53, 54], there are conflicting data about sex differences in autonomic dysfunction in PD. While some studies find that women are more likely to experience orthostatic hypotension [55, 56], a study looking at early PD (using a similar cohort to ours) found that men are more likely to experience orthostatic hypotension compared to women [57]. Additional research into potential sex-based differences in autonomic dysfunction in PD is warranted.
In summary, a subgroup of individuals with early PD had evidence of chronotropic incompetence during maximal cardiopulmonary exercise testing which may be secondary to cardiovascular autonomic dysfunction. Further studies should investigate the consequences of autonomic dysfunction on exercise tolerance and the effects of autonomic dysfunction on the potential benefit of exercise training in PD.
ACKNOWLEDGMENTS
The authors have no acknowledgments to report.
FUNDING
The Study in Parkinson’s Disease of Exercise (SPARX) trial was funded by grant NINDSR01 NS074343. Support for this paper was provided by U01 NS113851 from the National Institute of Neurologic Disease and Stroke.
CONFLICT OF INTEREST
The authors have no conflict of interest to report.
DATA AVAILABILITY
Data sharing is not applicable to this article as this is a secondary analysis, and no datasets were generated during this study.
SUPPLEMENTARY MATERIAL
[1] The supplementary material is available in the electronic version of this article: https://dx.doi.org/10.3233/JPD-230006.
REFERENCES
[1] | Kalia LV , Lang AE ((2015) ) Parkinson’s disease. Lancet 386: , 896–912. |
[2] | Coon EA ((2020) ) Autonomic dysfunction in the synucleinopathies. Semin Neurol 40: , 492–501. |
[3] | Merola A , Romagnolo A , Rosso M , Suri R , Berndt Z , Maule S , Lopiano L , Espay A ((2018) ) Autonomic dysfunction in Parkinson’s disease: A prospective cohort study. Mov Disord 33: , 391–397. |
[4] | Lamotte G , Lenka A ((2022) ) Orthostatic hypotension in Parkinson disease: What is new? Neurol Clin Pract 12: , e112–e115. |
[5] | Sabino-Carvalho JL , Fisher J , Vianna L ((2021) ) Autonomic function in patients with Parkinson’s disease: From rest to exercise. Front Physiol 12: , 626–640. |
[6] | Brubaker PH , Kitzman DW ((2011) ) Chronotropic incompetence: Causes, consequences, and management. Circulation 123: , 1010–1020. |
[7] | Schenkman M , Moore CG , Kohrt WM , Hall DA , Delitto A , Comella CL , Josbeno DA , Christiansen CL , Berman BD , Kluger BM , Melanson EL , Jain S , Robichaud JA , Poon C , Corcos DM ((2018) ) Effect of high-intensity treadmill exercise on motor symptoms in patients with de novo Parkinson disease: A phase 2 randomized clinical trial. JAMA Neurol 75: , 219–226. |
[8] | van der Kolk NM , de Vries NM , Kessels RPC , Joosten H , Zwinderman AH , Post B , Bloem BR ((2019) ) Effectiveness of home-based and remotely supervised aerobic exercise in Parkinson’s disease: A double-blind, randomised controlled trial. Lancet Neurol 18: , 998–1008. |
[9] | Johansson ME , Cameron IGM , Van der Kolk NM , de Vries NM , Klimars E , Toni I , Bloem BR , Helmich RC ((2022) ) Aerobic exercise alters brain function and structure in Parkinson’s disease: A randomized controlled trial. Ann Neurol 91: , 203–216. |
[10] | Mak MKY , Wong-Yu ISK ((2021) ) Six-month community-based brisk walking and balance exercise alleviates motor symptoms and promotes functions in people with Parkinson’s disease: A randomized controlled trial. J Parkinsons Dis 11: , 1431–1441. |
[11] | DiFrancisco-Donoghue J , Elokda A , Lamberg EM , Bono N , Werner WG ((2009) ) Norepinephrine and cardiovascular responses to maximal exercise in Parkinson’s disease on and off medication. Mov Disord 24: , 1773–1778. |
[12] | Palma JA , Carmona-Abellan MM , Barriobero N , Trevino-Peinado C , Garcia-Lopez M , Fernandez-Jarne E , Luquin MR ((2013) ) Is cardiac function impaired in premotor Parkinson’s disease? A retrospective cohort study. Mov Disord 28: , 591–596. |
[13] | Kanegusuku H , Silva-Batista C , Peçanha T , Nieuwboer A , Silva ND Jr. , Costa LA , de Mello MT , Piemonte ME , Ugrinowitsch C , Forjaz CL ((2016) ) Blunted maximal and submaximal responses to cardiopulmonary exercise tests in patients with Parkinson disease. Arch Phys Med Rehabil 97: , 720–725. |
[14] | Roberson KB , Signorile JF , Singer C , Jacobs KA , Eltoukhy M , Ruta N , Mazzei N , Buskard ANL ((2019) ) Hemodynamic responses to an exercise stress test in Parkinson’s disease patients without orthostatic hypotension. Appl Physiol Nutr Metab 44: , 751–758. |
[15] | Werner WG , DiFrancisco-Donoghue J , Lamberg EM ((2006) ) Cardiovascular response to treadmill testing in Parkinson disease. J Neurol Phys Ther 30: , 68–73. |
[16] | Fisher J ((2014) ) Autonomic control of the heart during exercise in humans: Role of skeletal muscle afferents. Exp Physiol 99: , 300–305. |
[17] | Goldstein D ((2014) ) Dysautonomia in Parkinson disease. Compr Physiol 4: , 805–826. |
[18] | De Pablo-Fernandez E , Tur C , Revesz T , Lees AJ , Holton JL , Warner TT ((2017) ) Association of autonomic dysfunction with disease progression and survival in Parkinson disease. JAMA Neurol 74: , 970–976. |
[19] | Merola A , Romagnolo A , Rosso M , Lopez-Castellanos J , Wissel B , Larkin S , Bernardini A , Zibetti M , Maule S , Lopiano L , Espay A ((2016) ) Orthostatic hypotension in Parkinson’s disease: Does it matter if asymptomatic? Parkinsonism Relat Disord 33: , 65–71. |
[20] | Moore CG , Schenkman M , Kohrt WM , Delitto A , Hall DA , Corcos D ((2013) ) Study in Parkinson disease of exercise (SPARX): Translating high-intensity exercise from animals to humans. Contemp Clin Trials 36: , 90–98. |
[21] | Hughes A , Daniel S , Kilford L , Lees A ((1992) ) Accuracy of clinical diagnosis of idiopathic Parkinson’s disease: A clinico-pathological study of 100 cases. J Neurol Neurosurg Psychiatry 55: , 181–184. |
[22] | Gardner AW , Skinner JS , Cantwell BW , Smith LK ((1991) ) Progressive vs single-stage treadmill tests for evaluation of claudication. Med Sci Sports Exerc 23: , 402–408. |
[23] | Fletcher GF , Ades PA , Kligfield P , Arena R , Balady GJ , Bittner VA , Coke LA , Fleg JL , Forman DE , Gerber TC , Gulati M , Madan K , Rhodes J , Thompson PD , Williams MA ((2013) ) Exercise standards for testing and training. Circulation 128: , 873–934. |
[24] | Tanaka H , Monahan KD , Seals DR ((2001) ) Age-predicted maximal heart rate revisited. J Am Coll Cardiol 37: , 153–156. |
[25] | Brubaker PH , Joo KC , Stewart KP , Fray B , Moore B , Kitzman DW ((2006) ) Chronotropic incompetence and its contribution to exercise intolerance in older heart failure patients. J Cardiopulm Rehab 26: , 86–89. |
[26] | Phan TT , Shivu GN , Abozguia K , Davies C , Nassimizadeh M , Jimenez D , Weaver R , Ahmed I , Frenneaux M ((2010) ) Impaired heart rate recovery and chronotropic incompetnce in patients with heart failure with preserved ejection fraction. Circ Heart Fail 3: , 29–34. |
[27] | van Twist D , Harms M , van Wijnen V , Claydon V , Freeman R , Cheshire W , Wieling W ((2021) ) Diagnostic criteria for initial orthostatic hypotension: A narrative review. Clin Auton Res 31: , 685–698. |
[28] | Wieling W , Kaufmann H , Claydon V , van Wijnen V , Harms M , Juraschek S , Thijs R ((2022) ) Diagnosis and treatment of orthostatic hypotension. Lancet Neurol 21: , 735–746. |
[29] | Sabino-Carvalho JL , Teixeira A , Samora M , Daher M , Vianna LC ((2018) ) Blunted cardiovascular responses to exercise in Parkinson’s disease patients: Role of the muscle metaboreflex. J Neurophysiol 120: , 1516–1524. |
[30] | Sabino-Carvalho JL , Vianna LC ((2020) ) Altered cardiorespiratory regulation during exercise in patients with Parkinson’s disease: A challenging non-motor feature. SAGE Open Med 8: , 2050312120921603. |
[31] | Imrich R , Eldadah BA , Bentho O , Pechnik S , Sharabi Y , Holmes C , Grossman E , Goldstein DS ((2009) ) Functional effects of cardiac sympathetic denervation in neurogenic orthostatic hypotension. Parkinsonism Relat Disord 15: , 122–127. |
[32] | Nakamura T , Hirayama M , Yamashita F , Uchida K , Hama T , Watanabe H , Sobue G ((2010) ) Lowered cardiac sympathetic nerve performance in response to exercise in Parkinson’s disease. Mov Disord 25: , 1183–1189. |
[33] | Fanciulli A , Kerer K , Leys F , Seppi K , Kaufmann H , Norcliffe-Kaufmann L , Wenning G ((2020) ) Validation of the neurogenic orthostatic hypotension ratio with active standing. Ann Neurol 88: , 643–645. |
[34] | Jain S , Ton T , Perera S , Zheng Y , Stein P , Thacker E , Strotmeyer E , AB N , Longstreth W Jr. ((2012) ) Cardiovascular physiology in pre-motor Parkinson disease: A neuroepidemiologic study. Mov Disord 27: , 988–995. |
[35] | Akbilgic O , Kamaleswaran R , Mohammed A , Ross G , Masaki K , Petrovitch H , Tanner C , Davis R , Goldman S ((2020) ) Electrocardiographic changes predate Parkinson’s disease onset. Sci Rep 10: , 11319. |
[36] | Kim J , Kim B , Koh S , Park K ((2014) ) Autonomic dysfunction according to disease progression in Parkinson’s disease. Parkinsonism Relat. Disord. 20: , 303–307. |
[37] | Reuter I , Engelhardt M , Freiwaldt J , Baas H ((1999) ) Exercise test in Parkinson’s disease. Clin Auton Res 9: , 129–134. |
[38] | Baschieri F , Sambati L , Guaraldi P , Barletta G , Cortelli P , Calandra-Buonaura G ((2021) ) Neurogenic orthostatic hypotension in early stage Parkinson’s disease: New insights from the first 105 patients of the BoProPark study. Parkinsonism Relat Disord 93: , 12–18. |
[39] | Fanciulli A , Campese N , Goebel G , Ndayisaba JP , Eschlboeck S , Kaindlstorfer C , Raccagni C , Granata R , Bonuccelli U , Ceravolo R , Seppi K , Poewe W , Wenning GK ((2020) ) Association of transient orthostatic hypotension with falls and syncope in patients with Parkinson disease. Neurology 95: , e2854–e2865. |
[40] | Rascol O , Perez-Lloret S , Damier P , Delval A , Derkinderen P , Destée A , Meissner WG , Tison F , Negre-Pages L ((2015) ) Falls in ambulatory non-demented patients with Parkinson’s disease. J Neural Transm (Vienna) 122: , 1447–1455. |
[41] | Horsager J , Andersen KB , Knudsen K , Skjærbæk C , Fedorova TD , Okkels N , Schaeffer E , Bonkat SK , Geday J , Otto M , Sommerauer M , Danielsen EH , Bech E , Kraft J , Munk OL , Hansen SD , Pavese N , Göder R , Brooks DJ , Berg D , Borghammer P ((2020) ) Brain-first versus body-first Parkinson’s disease: A multimodal imaging case-control study. Brain 143: , 3077–3088. |
[42] | Kujawa K , Leurgans S , Raman R , Blasucci L , Goetz C ((2000) ) Acute orthostatic hypotension when starting dopamine agonists in Parkinson’s disease. JAMA Neurol 57: , 1461–1463. |
[43] | Nimmons D , Bhanu C , Orlu M , Schrag A , Walters K ((2022) ) Orthostatic hypotension and antiparkinsonian drugs: A systematic review and meta-analysis. J Geriatr Psychiatry Neurol 35: , 639–654. |
[44] | Protas EJ , Stanley RK , Jankovic J , MacNeill B ((1996) ) Cardiovascular and metabolic responses to upper- and lower-extremity exercise in men with idiopathic Parkinson’s disease. Phys Ther 76: , 34–40. |
[45] | Wilmore J , Costill D ((1994) ) Physiology of Sport and Exercise, Human Kinetics Publishers Inc. |
[46] | Keteyian SJ , Brawner CA , Schairer JR , Levine TB , Levine AB , Rogers FJ , Goldstein S ((1999) ) Effects of exercise training on chronotropic incompetence in patients with heart failure. Am Heart J 138: , 233–240. |
[47] | Hales C , Servais J , Martin C , Kohen D ((2019) ) Prescription drug use among adults aged 40–79 in the United States and Canada. NCHS Data Brief, no 347. National Center for Health Statistics, Hyattsville, MD. |
[48] | Eston R , Connolly D ((1996) ) The use of ratings of perceived exertion for exercise prescription in patients receiving beta-blocker therapy. Sports Med 21: , 176–190. |
[49] | Magri D , Palermo P , Cauti F , Contini M , Farina S , Cattadori G , Apostolo A , Salvioni E , Magini A , Vignati C , Alimento M , Sciomer S , Bussotti M , Agostoni P ((2012) ) Chronotropic incompetence and functional capacity in chronic heart failure: No role of β-blockers and β-blocker dose. Cardiovasc Ther 30: , 100–108. |
[50] | Hirsh B , Mignatti A , Garan A , Uriel N , Colombo P , Sims D , Jorde U ((2012) ) Effect of β-blocker cessation on chronotropic incompetence and exercise tolerance in patients with advanced heart failure. Circ Heart Fail 5: , 560–565. |
[51] | Smart N , Clark H , Brubaker P , Witte K , Gierula J , Patel H , Pearson M ((2022) ) Effects of chronotropic incompetence on exercise capacity in people with heart failure versus age-matched controls. Heart Failure Rev 27: , 795–809. |
[52] | Ross RM ((2003) ) ATS/ACCP statement on cardiopulmonary exercise testing. Am J Respir Crit Care Med 167: , 1451; author reply 1451. |
[53] | Zirra A , Rao S , Bestwick J , Rajalingam R , Marras C , Blauwendraat C , Mata I , Noyce A ((2023) ) Gender differences in the prevalence of Parkinson’s disease. Mov Disord 10: , 86–93. |
[54] | Cerri S , Mus L , Blandini F ((2019) ) Parkinson’s disease in women and men: What’s the difference? J Parkinsons Dis 9: , 501–515. |
[55] | Georgiev D , Hamberg K , Hariz M , Forsgren L , Hariz G ((2017) ) Gender differences in Parkinson’s disease: A clinical perspective. Acta Neurol Scand 136: , 570–584. |
[56] | Kovács M , Makkos A , Aschermann Z , Janszky J , Komoly S , Weintraut R , Karádi K , Kovács N ((2016) ) Impact of sex on the nonmotor symptoms and the health-related quality of life in Parkinson’s disease. Parkinsons Dis 2016: , 7951840. |
[57] | Szewczyk-Krolikowski K , Tomlinson P , Nithi K , Wade-Martins R , Talbot K , Ben-Shlomo Y , Hu M ((2014) ) The influence of age and gender on motor and non-motor features of early Parkinson’s disease: Initial findings from the Oxford Parkinson Disease Center (OPDC) discovery cohort. Parkinsonism Relat Disord 20: , 99–105. |