Abstract
In this work, a new kind of YGdBa4CuNbOy (YGdNb-11411) nano-particles was designed and sintered using a solid-state reaction method, whereas their impact on the performance of GdBCO bulks was investigated for the first time. From the acquired x-ray diffraction data and scanning electron microscopy imaging results, it was proved that the YGdNb-11411 particles were of excellent chemical stability and did not react with other phases during the crystal growth process. The YGdNb-11411 particles ranged from 80 to 130 nm and they were uniformly distributed in the well-textured GdBCO bulk superconductors. The maximum critical current density (Jc-max) of the samples was enhanced from 3.48 × 104 A cm−2 to 8.63 × 104 A cm−2 (77 K) in the self-field as the doping amount increased from 0 wt% to 7 wt%. In addition, the GdBCO sample with YGdNb-11411 particles also showed an enhanced flux pinning force density and relatively high Jc around the magnetic flux density range of 1 T–2 T (77 K). These results clearly indicated that the YGdNb-11411 particles could effectively enhance the properties of the GdBCO bulk superconductors.
Export citation and abstract BibTeX RIS
1. Introduction
The REBa2Cu3O7−δ bulk superconductor is widely used in various applications due to its high critical temperature (Tc) and critical current density (Jc), which can trap and maintain high fields [1–3]. Therefore, REBCO bulk superconductors possess a wide range of potential applications, such as superconducting flywheel energy storage systems [1, 4], maglev systems [5, 6], high field permanent magnets [7–9], etc. To evaluate the performance of the REBCO bulk superconductor, the Jc is a crucial parameter. The Jc is determined by factors such as material type, temperature, and flux pinning center [10–12]. Among all, the addition of effective flux pinning centers to improve sample performance has always been a focus and hot topic of research.
Initially, the non-superconducting phase of the RE2BaCuO5 (RE211) particles with even tens of micro-size dimensions [13] was assumed to be effective flux pinning centers that formed during the peritectic reaction process. As a result, the superconducting phase is reduced, which negatively affects the sample performance. Various physical methods such as ball milling [14, 15], ultrasonic pre-treatment [16], and adjustment of the undercooling degree [17] have been developed to adjust the size of RE211 particles. However, the size of the RE211 particles remains in the micron or sub-micron range due to the Ostwald ripening effect. At the same time, this problem was also improved by chemical doping. More specifically, RE211 particles were adequately refined and the performance of REBCO superconductors was enhanced by doping with Pt [18, 19] and CeO2 [20–23]. According to a recently published work by Yang et al [24], the Gd211 particle size ranged only 1–3 μm in the sample doping with CeO2, while the nano-particles size was around 100–300 nm; the levitation force of the bulk with a diameter (d) 20 mm was above 54.5 N. Consequently, the incorporation of nano-particles can productively improve the properties of the bulk superconductor. However, the effective flux pinning force can be achieved only when the size of the particles is near the coherent length (ξab ≈ 2–4 nm below 77 K for REBCO bulk superconductors) [25–27]. To attain greater flux pinning performance, the RE2Ba4CuMOy (RE-2411) has been introduced in REBCO bulk superconductors as effective flux pinning centers [28–33]. Moreover, it has been partially demonstrated that the Y2Ba4CuNbOy (YNb2411) particles do not ripen during melt processing [34, 35]. The size of YNb2411 particles is between 250–400 nm [36, 37]. Babu et al found that the Jc value of the GdBCO bulk superconductor reached 5.5 × 104 A cm−2 by adding Gd2Ba4CuNbOy [30, 34, 38]. To compare the influence of RE211 and RE2411 on the properties of the samples, the SmBCO bulk superconductors with the initial compositions of [70%Sm123 + 30%Sm211 + 2%BaO2] and [70%Sm123 + 20%Sm211 + 10%Sm2411 + 2%BaO2], the Jc value of the samples with Sm2411 particles was 7.25 × 104 A cm−2, which was more than three times that of the samples that were only doped with Sm211 [32].
All the above-mentioned results indicated that the RE2Ba4CuMOy is one of the smaller non-superconducting particles, which exhibits excellent chemical stability and dose not react with other phases during the crystal growth process. As a result, the performance of the samples can be significantly improved. However, there is not any works on how to develop new ways to modify the properties of the RE2Ba4CuMOy phase, and its effect on the performance of the REBCO bulks, but this is of great significance and necessity. Under this perspective, in this work, a new kind of nanoscale YGdBa4CuNbOy powder was developed based on the Y2Ba4CuNbOy phase, and its influence on the performance of the GdBCO sample was thoroughly investigated.
2. Experimental
2.1. Preparation of YGdBa4CuNbOy powder
Precursor powders including BaCO3, Y2O3, Gd2O3, CuO, and Nb2O5 were used, all of which are commercial powders from China (Sinopharm Ltd) with a purity of 99%. The YGdBa4CuNbOy (YGdNb-11411) powder was sintered by the conventional solid-state reaction method. The powders of Y2O3, Gd2O5, BaCO3, CuO, and Nb2O5 were combined in a 1:1:8:2:1 molar ratio, described as follows:
Y2O3 + Gd2O3 + 8BaCO3 + 2CuO + Nb2O5 → 2YGdBa4 CuNbOy + 8CO2↑.
The powders were evenly mixed and sintered at various temperature,1050 °C, 1100 °C, 1150 °C, 1200 °C, and 1250 °C for 24 h.
2.2. Preparation of the GdBCO bulk superconductor
The GdBCO bulk superconductors were made using the top-seeded infiltration growth (TSIG) [39] method. Particularly, the solid phase powders with different addition of YGdNb-11411 [(99−x)wt% (Gd2O3 + 1.2BaCuO2) + xwt% YGdNb-11411 + 1 wt% CeO2 (x = 0, 3, 5, 7, 10, and 15)] were individually pressed into pellets (d = 32 mm, 35 g). At the same time, the liquid phase [10BaCuO2 + 6CuO + Y2O3] and Y2O3 powders were uniaxially pressed into precursor pellets (d = 40 mm). The Y2O3 substrate pellet served as the support substrate. The weight of the liquid phase was 1.4 times that of the solid phase. Along the coaxial line, the solid precursor pellet was placed on the liquid precursor pellet [40]. An NdBCO seed was put by its ab-plane on the top of the solid precursor pellet to induce the development of the precursor bulk. All precursor bulks were put into a high-temperature furnace and completed crystal growth with the same heat-treatment procedure [40]. Finally, by carrying out oxygenation processes [40], the GdBCO precursor bulks could complete the phase transition and become superconductor bulk.
Citing as an example, the morphology of the GdBCO bulk superconductor with 7 wt% YGdNb-11411 is shown in figure 1. As can be observed, the sample has a clear cross pattern, indicating that it is a single-domain GdBCO bulk superconductor.
Figure 1. Morphology of the bulk GdBCO superconductor with 7 wt% YGdNb-11411 addition.
Download figure:
Standard image High-resolution image2.3. Measurement details
The samples are 5 mm away from the seeds at the growth sector. First, the bulk superconductors with different YGdNb-11411 addition are cut into cuboid with 2 × 3 × 10 mm3 along it's a–c plane by diamond wire cutting machine (STX-202A, China). Then, the samples with a size 2 × 3 × 2 mm3 were obtained by natural cleavage from cuboid bulk superconductor along the a–b plane for carrying out the magnetic property measurements. The detailed information of the sample is shown in table 1. The growth region of the samples with varying concentrations of YGdNb-11411 particle inclusions was grounded into powder for conducting the x-ray diffraction (XRD) (Model Rigaku D/max 2550X, Japan) measurements. The microstructure of the samples was measured by scanning electron microscopy (SEM, Nava Nano SEM 450, Czech Re-public). Moreover, the magnetic hysteresis loops and the Tc of the samples with varying amounts of YGdNb-11411 particle inclusions were measured using a vibrating sample magnetometer (VSM, CFM-8T, United Kingdom), while Jc was calculated by the modified Bean model [41] with the following formula:
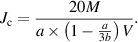
Table 1. The detailed information of the sample.
Sample | S1 | S2 | S3 | S4 | S5 | S6 |
---|---|---|---|---|---|---|
YGNb-11411 doping amount (wt%) | 0 | 3 | 5 | 7 | 10 | 15 |
Sample size (mm3) | 2 × 3 × 2 |
Among them, M (emu) is the difference of magnetic moments on the magnetic hysteresis loop branches for the decreasing and increasing magnetic field, respectively; a (mm) and b (mm) (a < b) are the sample dimensions on its plane perpendicular to the magnetic field direction, and V (mm3) represents the volume of the sample.
3. Results and discussion
The acquired XRD pattern of the YGdNb-11411 powders sintered under different temperatures is displayed in figure 2. The YGdNb-11411 (PDF#24–1024) phase can be observed when they were sintered under different temperatures, as shown in figure 2. The microstructures of the five types of powder were also observed using SEM, as illustrated in figure 3. The YGdNb-11411 phase aggregated when the temperature was lower, such as 1050 °C and 1100 °C, as can be seen in figures 3(a) and (b). However, the particles got coarser and tend to range in size from 200 to 800 nm when the sintering temperature was raised, as illustrated in figures 3(c)–(f). As a result, the smallest YGdNb-11411 particle size was about 200–300 nm, obtained when the powder was sintered at 1150 °C.
Figure 2. The XRD spectra of the YGdNb-11411 powder sintered under different temperatures.
Download figure:
Standard image High-resolution imageFigure 3. The SEM images of the YGdNb-11411 powder sintered under different temperature conditions. (a) 1050 °C, (b) 1100 °C, (c) 1150 °C, (d) 1200 °C, and (e) 1250 °C.
Download figure:
Standard image High-resolution imageThe Jc of the samples is illustrated in figure 4. The Jc-max of the GdBCO specimen increased from 3.48 × 104 A cm−2 (sample S1) to 8.63 × 104 A cm−2 (sample S4) in the self-field as the doping amount increased from 0 wt% to 7 wt%, as shown in the insert of figure 4. Then, the Jc-max decreased from 8.63 × 104 A cm−2 (sample S4) to 2.62 × 104 A cm−2 (sample S6) in the self-field as the doping amount increased from 7 wt% to 15 wt% as shown in the insert of figure 4 and table 2. The largest self-field Jc-max value was reached when the YGdNb-11411 amount was 7 wt%. Simultaneously, it can be clearly seen that the fishtail effect for samples was significantly enhanced with the increase in the doping amount to 7 wt%, which means that the sample has a good current carrying capacity at moderate fields. Then, the fishtail effect gradually weakened with the increase in the doping amount.
Figure 4. The Jc–B curves of the samples at 77 K. The maximum critical current density as a function of the doping amount of YGdNb-11411 is shown in the inset.
Download figure:
Standard image High-resolution imageTable 2. The properties of GdBCO samples with different addition of YGdNb-11411.
Sample | S1 | S2 | S3 | S4 | S5 | S6 |
---|---|---|---|---|---|---|
Jc (104 A cm−2) | 3.48 | 3.76 | 5.16 | 8.64 | 3.12 | 2.61 |
Fp (N m−2) | 1.78 | 3.01 | 5.21 | 8.66 | 1.94 | 1.17 |
Bp (T) | 1.25 | 1.35 | 1.65 | 2.15 | 1.15 | 0.95 |
b = B/Birr | 0.27 | 0.28 | 0.34 | 0.42 | 0.34 | 0.33 |
The flux pinning force density (Fp) of the samples under different magnetic fields was calculated according to the formula Fp = Jc × B, as shown in figure 5(a). The variation tendencies of Fp–B curves showed that the flux pinning force density was increased first and then decreased by increasing the applied field B from 0 to 5 T for all the samples. Nonetheless, the Fp values were much different in two respects: (1) the Fp began to increase and then decrease when the amount of YGdNb-11411 x increased. The maximum Fp (Fp-max) of the sample S4 with 7 wt% YGdNb-11 411 was about 8.66 N m−2, which was more than 4.8 times that of undoped sample S1; (2) the peaks of Fp–B curves for the GdBCO samples were also shifted to higher field values first and then fall to lower field values when the amount of YGdNb-11411 x increased. The magnetic flux density where the peak occurred is denoted by Bp, corresponding to the Fp-max. The Bp of the GdBCO samples began to increase and then decrease when the amount of YGdNb-11411 x increased. These results indicated that the Fp was greatly improved in GdBCO bulk with appropriate YGdNb-11411 additions, such as the Fp-max value of the GdBCO samples was greatly enhanced from 1.78 N m−2 to 8.66 N m−2 when the YGdNb-11411 additions increased from 0 wt% to 7 wt% as shown in table 2. The magnetic field Bp, corresponding to the maximum flux pinning force (Fp-max) of the GdBCO samples was also improved to a higher field, such as the Bp value of the GdBCO samples was greatly enhanced from 1.25 T to 2.15 T when the YGdNb-11411 additions increased from 0 wt% to 7 wt% as shown in table 2. The change in the Fp and the Bp indicate that the magnetic flux pinning center of the GdBCO bulk superconductors was altered after doping YGdNb-11411 particles.
Figure 5. (a) Depiction of the variation curves of the Fp along with the magnetic field. (b) The curves of the relationship between f and b at 77 K.
Download figure:
Standard image High-resolution imageIn order to understand the magnetic flux pinning mechanism of the sample, the relationship between the reduced field b (b = B/Birr, Birr being irreversibility field) and f (f= Fp/Fp-max) [42] was analyzed as shown in figure 5(b). The results show that the b(fp) corresponding to the peak value fp of the sample increased from 0.27 to 0.42 with the doping amount of YGdNb-11411 increased from 0 wt% to 7 wt%, as shown in table 2. According to the Dew Hughes model and related research [43–45], the maximum value of b(fp) is less than 0.5 corresponding to the Δl-type strong pinning force. These indicates that the YGdNb-11411 nano-particles is of Δl-type strong pinning centers, and they can really enhance the magnetic flux pinning force and the physical properties of the samples. This outcome will further confirm the magnetic flux pinning mechanism of the GdBCO bulk superconductors by analyzing Tc, chemical components, and microstructure.
As illustrated in figure 6, the Tc values of the GdBCO bulk superconductors with various amounts of YGdNb-11411 particle inclusions were almost constant at 92 ± 1 K and exhibited a narrow superconductor transition width (ΔT ≈ 3.5 K). Therefore, the GdBCO bulk had strong inter-grain connectivity due to the small superconductor transition width and there was no obvious substitution of the Y–Nb element occurred within the Gd-123 matrix.
Figure 6. The temperature-dependent normalized magnetic moment curve showing the Tc of the samples.
Download figure:
Standard image High-resolution imageTo further confirm this result, the chemical composition of the YGdNb-11411-doped GdBCO samples was analyzed. The growth region of the samples with various amounts of YGdNb-11411 particle inclusions was grounded and then the XRD spectra were recorded, as shown in figure 7. The dominant XRD diffraction peaks were Gd123 (PDF#86–0474) and Gd211(PDF#75–2427) phases. Simultaneously, the visible main diffraction peaks of the YGdNb-11411 (PDF#24–1024) phase gradually enhances with the increase of doping amount, but their positions are the same and without any shifting, which proves that the YGdNb-11411 phase exists stably in the GdBCO bulk superconductors. However, no distinct diffraction major peaks of the YGdNb-11411 phase were detected about the ground powder of sample S2 with 3 wt% YGdNb-11411, as can be ascertained in figure 7. This is probably because the fact that the YGdNb-11411 content was lower than that of the accuracy of XRD equipment. In addition, it is easy to see that the other peaks of YGdNb-11411 phase are not obvious compared to the main diffraction peak, which may be related to the crystal orientation of the particles, or the weak intensity compared to Gd123 and Gd211 phases. As a result, according to the b (0.27–0.42), Tc and XRD, it can be determined that doping YGdNb-11411 nano-particles provides effective Δl-type magnetic flux pinning centers for the bulk superconductor. This type of magnetic flux pinning center does not cause changes in lattice parameters. These results indicated that the YGdNb-11411 phase is of excellent chemical stability and does not react with the other phases during the crystal growth process.
Figure 7. The XRD of the ground powder of the GdBCO bulk superconductors.
Download figure:
Standard image High-resolution imageTherefore, to further explore the influence of the YGdNb-11411 phase on the GdBCO bulks, the microstructure of the samples was systematically analyzed using SEM imaging, as shown in figure 8. Three main types of particles were distributed on the Gd123 matrix. Type Ⅰ refers to the Gd211 particles in the range of 0.5 μm–2 μm, which were uniformly distributed in the sample. Type Ⅱ denotes needle-like YGdNb-11411 particles in a range of 80 nm–130 nm. These particles were nearly of the same shape and size, and were not closely related to the content of the YGdNb-11411 additions, as depicted in figure 8. However, the content of the YGdNb-11411 particles was simultaneously increased when the doping amount was increased from 3 wt% to 15 wt%. As illustrated in figure 8(d), both Gd211and YGdNb-11411 particles are evenly dispersed in the Gd123 matrix when the doping amount was 7 wt%. Type Ⅲ represents the irregularly shaped particles with sizes ranging from 1 μm to 3 μm. The irregularly shaped particles mainly appeared in the GdBCO samples with the YGdNb-11411 addition larger than 7 wt%, as can be observed in figures 8(e) and (f). The irregularly shaped particles were mainly accumulated by the YGdNb-11411, Gd123 and Gd211 phases. The proportion of the superconducting phase of the samples was reduced by the larger size irregularly shaped particles, especially in the samples with high YGdNb-11411 additions, and finally result in the reduction of Jc when x increased from 7 wt% to 15 wt%.
Figure 8. The SEM images of the samples with different addition of YGdNb-11411. (a) x = 0 wt%; (b) x = 3 wt%; (c) x = 5 wt%; (d) x = 7 wt%; (e) x = 10 wt%; (f) x = 15 wt%. Type-Ⅰ (yellow-color): Gd211 particles, Type-Ⅱ (pink-color): YGdNb-11411 particles, and type-Ⅲ (green-color): irregularly shaped particles.
Download figure:
Standard image High-resolution imageThe new nano-sized YGdNb-11411 particles were well introduced into the GdBCO bulks, and can play the role of effective magnetic flux pinning centers to enhance the physical properties of the samples. Consequently, the Jc-max value of the GdBCO specimen increased from 3.48 × 104 A cm−2 to 8.63 × 104 A cm−2 in the self-field as the YGdNb-11411 doping amount x increased from 0 wt% to 7 wt%, as shown in the insert of figure 4. Additionally, the Fp-max value of the GdBCO samples was greatly enhanced from 1.78 N m−2 to 8.66 N m−2; and the Bp value of the GdBCO samples was greatly enhanced from 1.25 T to 2.15 T at 77 K.
4. Conclusions
In summary, high-purity YGdNb-11411 particles of 200–300 nm were obtained by using the solid sintering reaction method at 1150 °C. Then, single-domain GdBCO bulk superconductors with varying amounts of YGdNb-11411 content were successfully prepared by Gd + 011 TSIG. From our experimental results, it was demonstrated that Jc of the samples was increased by increasing of the YGdNb-11411 addition from 0 wt% to 7 wt% and decreased when x was greater than 7 wt%. The Fp-max value of the GdBCO samples was greatly enhanced from 1.78 N m−2 to 8.66 N m−2; and the Bp value of the GdBCO samples was greatly enhanced from 1.25 T to 2.15 T. However, from the b (0.27–0.42), Tc and XRD results, it was proved that the doping of the YGdNb-11411 phase have no effect on the Gd123 phase. As a consequence, an appropriate doping amount of YGdNb-11411 nano-particles can used for creating effective magnetic flux pinning centers, enhancing the flux pinning force.
Acknowledgments
This work was supported by the National Natural Science Foundation in China (No. 52072229, No. 51572164), the Key Grant Project of Chinese Ministry of Education (No. 311033).
Data availability statement
All data that support the findings of this study are included within the article (and any supplementary files).