Scientific contributions and lessons learned from 30 years of ecological monitoring of the Bylot Island tundra ecosystem
- 1Département de Biologie and Centre d’études Nordiques, Université Laval, Québec, QC, Canada
- 2Chaire de Recherche du Canada en Biodiversité Nordique, Centre d’études Nordiques and Centre de la Science de la Biodiversité du Québec, Université du Québec à Rimouski, Rimouski, QC, Canada
- 3Centre for Arctic Knowledge and Exploration, Canadian Museum of Nature, Ottawa, ON, Canada
- 4Département de Biologie, Centre d’études Nordiques and Centre de la Science de la Biodiversité du Québec, Université de Sherbrooke, Sherbrooke, QC, Canada
The Arctic tundra has a relatively low biodiversity but species living there have unique adaptations and are exposed to unprecedented rates of climate warming. Monitoring changes in Arctic biodiversity and identifying the driving forces is thus a pressing issue. Bylot Island in the Canadian Arctic has one of the longest and most comprehensive monitoring programs of the tundra food web, spanning four decades. We provide a historical overview of ecological studies on Bylot Island, summarize their key scientific contributions, show their impacts, and present the ingredients for the success of the program and the main challenges encountered. Some major contributions include demonstrating the key role of predation in structuring the tundra food web, the importance of exchanges between ecosystems for the persistence of top predators and their cascading effects on trophic interactions, the apparent resistance of the vertebrate biota to climate warming, the need to consider multiple hypotheses to explain northward range expansion of species and the benefits of integrating scientific data and local knowledge into ecological monitoring. The program has produced >250 journal articles and >80 graduate student theses, which generated >7,700 citations in the scientific literature. A high proportion (65%) of the articles had more citations than comparable publications in their field. The longevity and success of the program can be attributed to several factors, including a researcher-driven (i.e. bottom-up) approach to design the monitoring; long-term commitment of a small number of dedicated researchers and the strong participation of graduate students; the adoption of a food web rather than a single species perspective; extensive presence in the field; the combination of several methodological approaches; and the use of multiple spatial scales adapted to research questions of interest. Challenges encountered include funding issues, transfer of expertise over time, limited spatial replication, statistical design and maintaining partnerships. Robust monitoring is essential to provide sound baseline to detect future changes, and lessons learned from our program could improve future monitoring schemes in the Arctic. Paradoxically, we believe that ecological monitoring on Bylot Island has been successful in large part because it was not originally designed as a monitoring program per se.
1 Introduction
The Arctic tundra is still largely a pristine environment although it is increasingly exposed to anthropogenic pressure such as industrial development, contaminants, or climate warming (Tolvanen et al., 2019; Aronsson et al., 2021). This biome is inhabited by relatively few living species in comparison to other biomes such as the tropical or even temperate forests (Willig et al., 2003, Willig et al., 2006). However, its biota presents adaptions that are quite unique on Earth due to the harsh prevailing conditions such as long periods of darkness, very cold winter temperatures, and lack of summer heat. Climate warming poses unique threats to Arctic species because, unlike other biomes that could be displaced toward the poles as the Earth’s climate warms, the Arctic tundra will simply shrink as it cannot be displaced further north (Rew et al., 2020). Monitoring changes in Arctic biodiversity and identifying the driving forces for observed changes is thus a pressing issue recognized by many international bodies such as the Arctic Council (Christensen et al., 2013; Meltofte, 2013).
Monitoring and understanding ecological changes taking place in the Arctic tundra is difficult because it remains one of the least known biomes to Western science. Remoteness, harsh climate, and the high costs of accessing and working in the Arctic have historically been major impediments to conducting field studies in this region, and they still are today to a large extent (Mallory et al., 2018). It is partly to address these problems and increase scientific knowledge of the Arctic region that the first International Polar Year (IPY), a large, internationally coordinated research effort, was instigated in 1882-83, and repeated periodically afterwards (in 1932-1933, 1957-1958 and 2007-2008; Barr and Lüdecke, 2010). These large research initiatives have yielded an enormous amount of new knowledge on the state, structure and functioning of Arctic ecosystems at a large spatial scale (Ferguson et al., 2012; Meltofte, 2013; Legagneux et al., 2014). Although IPYs may provide sound baseline data at multiple sites, they only provide a snapshot of the state of Arctic ecosystems and they are too far apart to really track changes occurring in them. Moreover, punctual observations are likely to miss stochastic events including those at the extreme specter of natural variability or occurring suddenly, which may still have long-lasting consequences. Evaluating how and why Arctic species, and in particular vertebrate ones, change over time requires sustained field monitoring at much finer temporal and spatial resolutions.
Ecological studies began on Bylot Island, Nunavut, in the early 1990s and they have become over time one of the longest monitoring programs of the tundra food web in the Arctic still running (Gauthier et al., 2013). It is now well known among the community of Arctic scientists and it has inspired other ecological monitoring programs in Canada and in other countries, such as those of the Canadian High Arctic Research Station and of the COAT initiative in Norway (Ims et al., 2013). In this paper, we present an overview of ecological studies performed on Bylot Island and how they evolved over four decades. We show their key scientific achievements, their impact in the field of Arctic ecology, and their contributions to Arctic biodiversity monitoring and government policies. We also identify the ingredients that led to the success of the program but also its challenges, which allows us to share lessons learned from our experience that could be useful for other monitoring programs.
2 Bylot Island ecosystem
Bylot Island is located at the northern tip of Baffin Island, between 72.7° and 73.8° N of latitude, at the entrance of the Northwest Passage in the Canadian Arctic. Most of the 11,100 km2 island is covered by mountains, a large ice cap and glaciers except for its southern portion where a 1,600 km2 rolling plain, mostly from 0-300 m ASL, is set between the mountains and the sea (Gauthier et al., 2011). The south plain has a relatively mild climate for the latitude due to its southern exposure and the presence of high mountains that partially block cold northerly winds. It is truly a polar oasis covered by lush tundra vegetation with 161 species of vascular plants and 133 species of bryophytes identified (https://bylot.cen.ulaval.ca/en/specieslist.php; Line Rochefort, unpubl. data). It also harbors a rich fauna by Arctic standards with 66 species of birds, 19 species of mammals (including the surrounding marine environment), and 38 families of arthropods (Lepage et al., 1998; Bolduc et al., 2013; Gauthier et al., 2023). Two prominent features of the biota are the presence of the largest breeding colony of greater snow geese (Anser caerulescens atlanticus; ca 25,000 pairs, Reed et al., 2002) on the south plan and seabird colonies on cliffs bordering the island (ca 150,000 pairs, Gaston et al., 2017). The high biodiversity of the island has long been recognized as it was designated a Migratory Bird Sanctuary in 1965 and was included in Sirmilik National Park at the time of its creation in 1999.
Bylot Island is located in bioclimatic zone C of Walker et al. (2005). The food web of the island tundra was described by Gauthier et al. (2011). The terrestrial vertebrate component is dominated by migratory birds, especially waterfowl, shorebirds, raptors and seabirds with only a handful of year-round resident species (Figure 1). Lemmings (Lemmus trimucronatus and Dicrostonyx groenlandicus), Arctic foxes (Vulpes lagopus) and ermines (Mustela richardsoni) are the main resident species. Geese, lemmings and to a much lower extent Arctic hares (Lepus arcticus) and rock ptarmigans (Lagopus muta) are the main herbivores. Large mammalian herbivores are either absent (Muskox, Ovibos moschatus) or rare visitors (caribou, Rangifer tarandus), as is their main predator, wolves (Canis lupus). The presence of a large contingent of migratory species implies that the tundra food web is radically different during the summer compared to the rest of the year (Figure 1). More importantly, migratory species create numerous connections between the tundra and more distant ecosystems around the globe, effectively creating a meta-ecosystem (Moisan et al., 2023; see below).
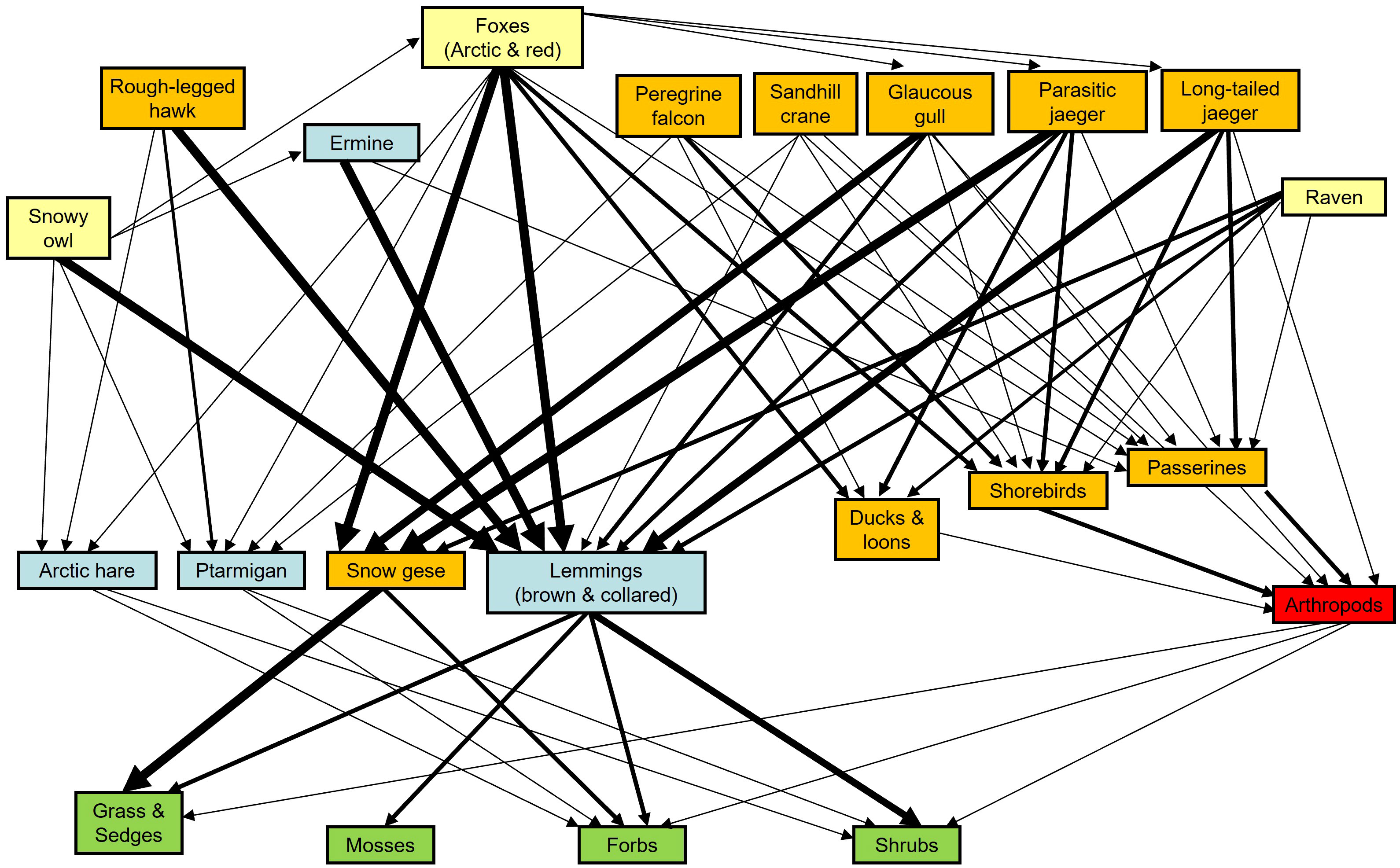
Figure 1 Bylot Island food web illustrated using five functional groups: migrants (orange), partial migrants (yellow), residents (blue), residents frozen in the soil during winter (red) and plants (green). Line thickness indicates relative strength of interactions between species (modified from Gauthier et al., 2011).
3 History of ecological studies on Bylot Island
The monitoring program of Bylot Island emerged from the growing commitment of researchers who initiated the first field studies in the late 1980s. Field work at the site started in 1989 by G. Gauthier from Université Laval and A. Reed from the Canadian Wildlife Service. The initial objectives of these studies were to determine the nutrient allocation strategy of breeding greater snow geese and evaluate the impact of goose grazing on Arctic vegetation (Gauthier et al., 2023). Destruction of wetland habitats by overabundant goose populations had already been reported at subarctic colonies, and this was emerging as a source of concern for the conservation of the Arctic tundra in several parts of North America (Kerbes et al., 1990; Ankney, 1996). Therefore, snow goose population dynamics and goose-plant interactions were important research topics initially, in part because of management issues (goose overgrazing impact) but also because the predominant view at the time was that plant-herbivore interactions dominated trophic interactions in the Arctic (see below). Snow geese and their food plants (graminoids) were thus key components monitored right from the start (Figure 2). Automated stations monitoring weather variables year-round were also implemented early in the project and expanded over time (CEN, 2022).
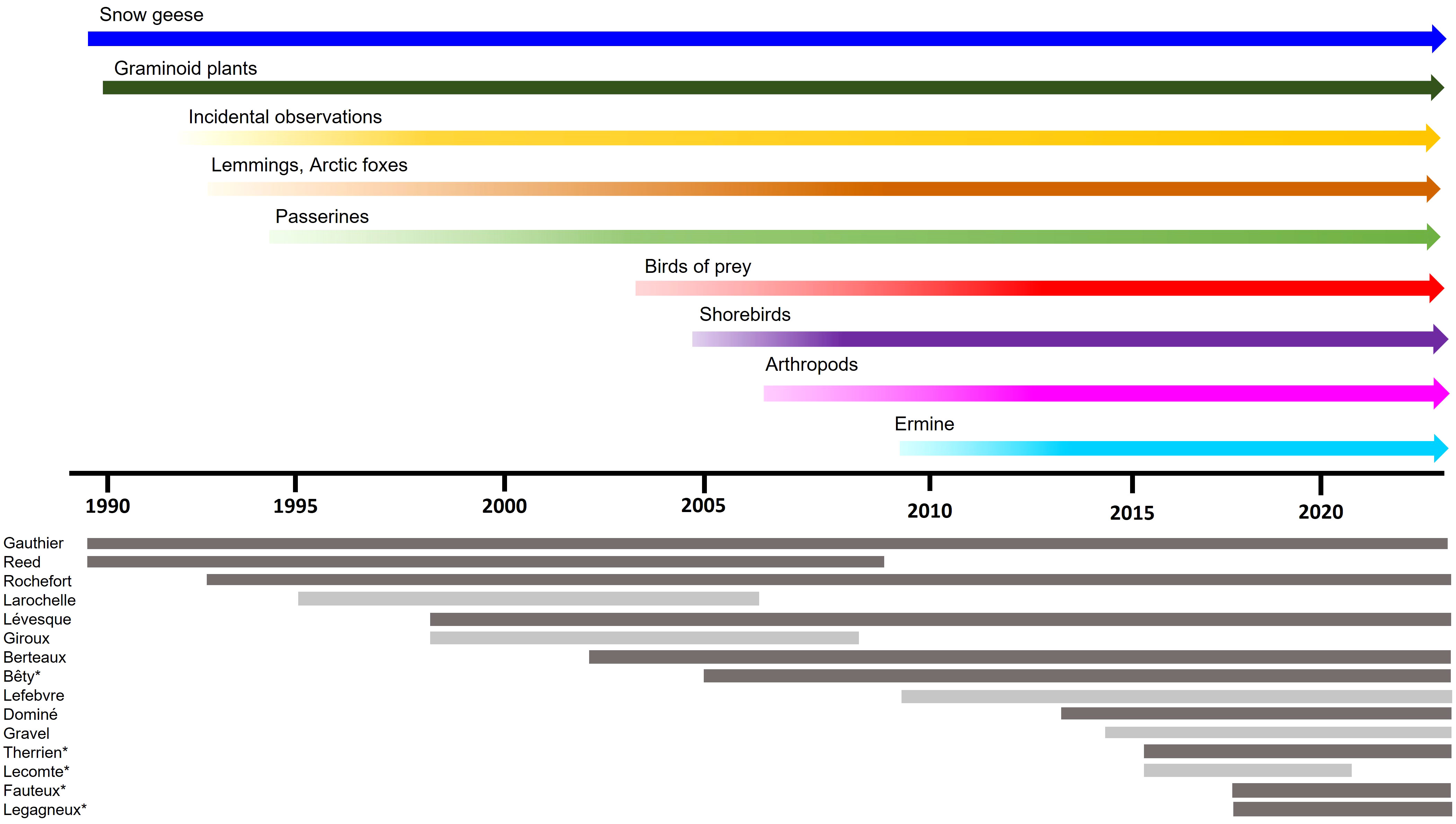
Figure 2 Biotic components of the tundra food web of Bylot Island monitored and researchers involved in the program over time. Dark grey: main researchers, light grey: other researchers. Stars (*) indicate researchers who completed graduate studies at Bylot Island.
After a few years, it became apparent that predation was also an important interaction in this food web. For instance, we showed that goose nesting success and productivity were strongly impacted by annual variations in predation pressure on nests (Lepage et al., 1996; Bêty et al., 2002; Morrissette et al., 2010). This unveiled complex and poorly known interactions between geese, predators and alternative or incidental prey in the food web. New studies were initiated on these subjects, which led to a reorientation of the project toward the study of predator-prey interactions. Monitoring of other functional groups were gradually added, including lemmings, Arctic foxes, passerines, birds of prey, shorebirds, arthropods and most recently ermines (Figure 2). This reorientation and expansion contributed to the emergence of a food web approach, which became the conceptual model of the program in the early 2000s (Figure 1). Understanding the mechanisms driving trophic interactions and whether the tundra food web was controlled by top-down (i.e. predators) or bottom-up (i.e. primary production) forces became overarching questions for our group (Legagneux et al., 2012).
Several researchers from various domains joined the team over the years, which strengthened the program and contributed to its expansion to the whole tundra food web (Figure 2). Additional expertise of people who played a key role in the long-term studies included ecologists specialized on various vertebrate groups (D. Berteaux, J. Bêty, J.F. Therrien, D. Fauteux and P. Legagneux), plant ecologists (L. Rochefort and E. Lévesque), a snow specialist (F. Domine) and a modeller (D. Gravel). It is noteworthy that several researchers who joined the team in recent years are former graduate students or postdoctoral fellows trained by researchers who initiated and led the program. Most investigators involved in the project over the years held academic positions at universities except for two at the Canadian Wildlife Service, one at the Canadian Museum of Nature and one at a private research center in the USA.
Our research program has primarily focused on vertebrate and, to a lesser extent, plant species. This largely reflects the primary interests of lead researchers who became committed to the program in the long-term (Gauthier et al., 2023). Nonetheless, we were successful in attracting and collaborating with other researchers who conducted numerous short-term studies on other components of the ecosystem such as the permafrost dynamics (e.g. Godin et al., 2016), soil processes (e.g. Deschamps et al., 2023), biogeochemical cycles in ponds (e.g. Préskienis et al., 2021), and snow physical properties (e.g. Domine et al., 2018a). Even though these additional components were not formally integrated to our core monitoring program, they contributed to expand our work and address original questions at the interface between disciplines (e.g. Beardsell et al., 2017; Domine et al., 2018b). More information on the history of the ecological monitoring program of Bylot Island can be found in Gauthier et al. (2023).
4 Scientific productivity of ecological studies on Bylot Island
We compiled all publications originating entirely or partly from our studies on Bylot Island and coauthored by at least one of us. From 1991 to August 2023, the program generated 253 peer-reviewed journal articles, 30 reports, book chapters or manuals, 24 PhD theses, and 56 MSc theses. Early-career researchers made a huge contribution to the publication record as 73% of journal articles had a student or post-doc as first author. Productivity has increased steadily over time, averaging 2.7 articles per year in the 1990s, 6.8 in the 2000s, 10.4 in the 2010s and 14.3 since 2020 (Figure 3). These publications fell mostly in the category fundamental research (67%), followed by methodological papers (31%), contributions to national/international projects or syntheses (31%) and applied research (13%) (see Supplementary Material for a definition of those categories). Only two articles (Gauthier et al., 2013, Gauthier et al., 2023) were primarily focused on documenting species status or trend with our monitoring data while one methodological paper used the monitoring of a focal species (Arctic fox) on Bylot Island as a starting point to harmonize monitoring activities at the circumpolar level (Berteaux et al., 2017).
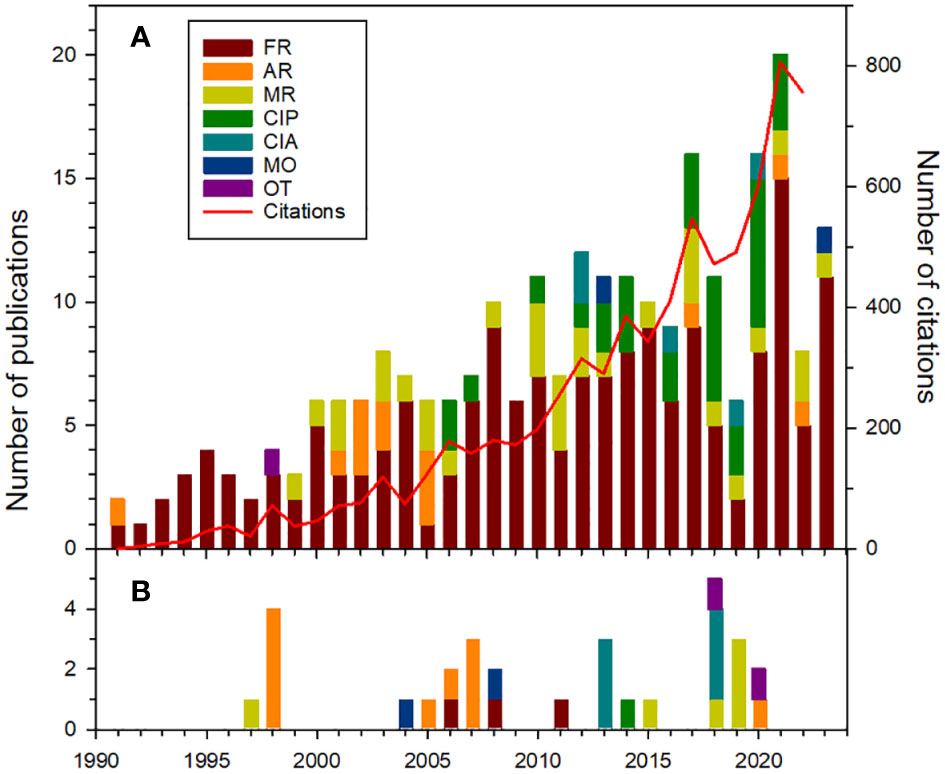
Figure 3 Annual number of articles in peer-reviewed journals and of citations (A) and annual number of reports and book chapters (B) from the Bylot Island ecological studies (as of 23 August 2023). FR, Fundamental research; AR, Applied research; MR, Methodological research; MO, Monitoring; CIP, Contribution to international projects; CIA, Contribution to international assessment; OT, others. See Supplementary Material for a full definition of these categories. Source for number of citations: Web of Science.
It is noteworthy that even though monitoring was at the heart of our long-term program since the beginning, papers specifically reporting status or tends using monitoring data were rare. Paradoxically, we believe that ecological monitoring on Bylot Island has been successful in large part because it was not designed as a monitoring program per se. In other words, monitoring was a foundation rather than an objective of our research program. Since the beginning, our work was always question-driven and usually structured in short-term (2 to 3-year) studies suitable for graduate student theses. Nonetheless, most of these studies fit within the long-term perspective of the program and usually address one or several boxes or arrows in our food web model (Figure 1). In doing so, we ensured that each student participated to the collection of a core set of monitoring data (e.g., annual abundance, reproductive effort and success) for key species of the food web. A significant proportion of our peer-reviewed articles (37%, n = 253) still relied on long-term data generated by the program (typically, data not acquired by graduate students during the course of their degree). Not surprisingly, the proportion of publications that include long-term monitoring data increased over time (0% in the 1990s, 34% in the 2000s, 38% in the 2010s and 54% since 2020). The incorporation of long-term data in publications addressing specific ecological questions undoubtedly strengthened these papers and rendered their conclusions more robust. In summary, monitoring is like the foundation of a house: you don’t see it once the house is built, but this is a key part of the building, and it is better to keep it in good order.
A bibliometric map based on the co-occurrence of terms (used as a surrogate of keywords) appearing in the title and abstract of our journal articles illustrates well the themes of ecological studies on Bylot Island and how they evolved over time (Figure 4; see methodological details in Supplementary Material). The analysis retained 267 terms that had between 2 and 122 occurrences (average: 14, median: 8) and between 17 and 256 links (i.e., co-occurrences; average: 92) with other terms in our articles. We could identify 3 major themes in our research activities based on this analysis. The first one is centered on population dynamics and includes terms like Arctic (122 occurrences), population (71), birds (54), phenology (44), survival (43), reproduction (40) and migration (38) (red circles on Figure 4). The second one focuses on plant-herbivore interactions and includes terms like snow goose (107 occurrences), young (52), climate change (42), growth (27), food (27), plant (24) and nitrogen (19) (green circles on Figure 4). The last one deals with predator-prey interactions and includes terms like predator (60 occurrences), Arctic fox (55), lemmings (54), nest (39), prey (36), population cycles (20) and trophic interactions (15) (blue circles on Figure 4). The temporal evolution of research themes can also be seen when examining when terms appeared most frequently in our publications. Terms related to plant-herbivore interactions were dominant in early years of the program (purple circles on Supplementary Figure S1) whereas those related to population dynamics and predator-prey interactions were dominant in more recent years (green and yellow circles on Supplementary Figure S1). Therefore, our publications reflect well the evolution of the project over time (see History of ecological studies). It is also interesting to note that the term “monitoring” was not among the most frequently used ones in this network analysis (14 occurrences in the blue circles, Figure 4).
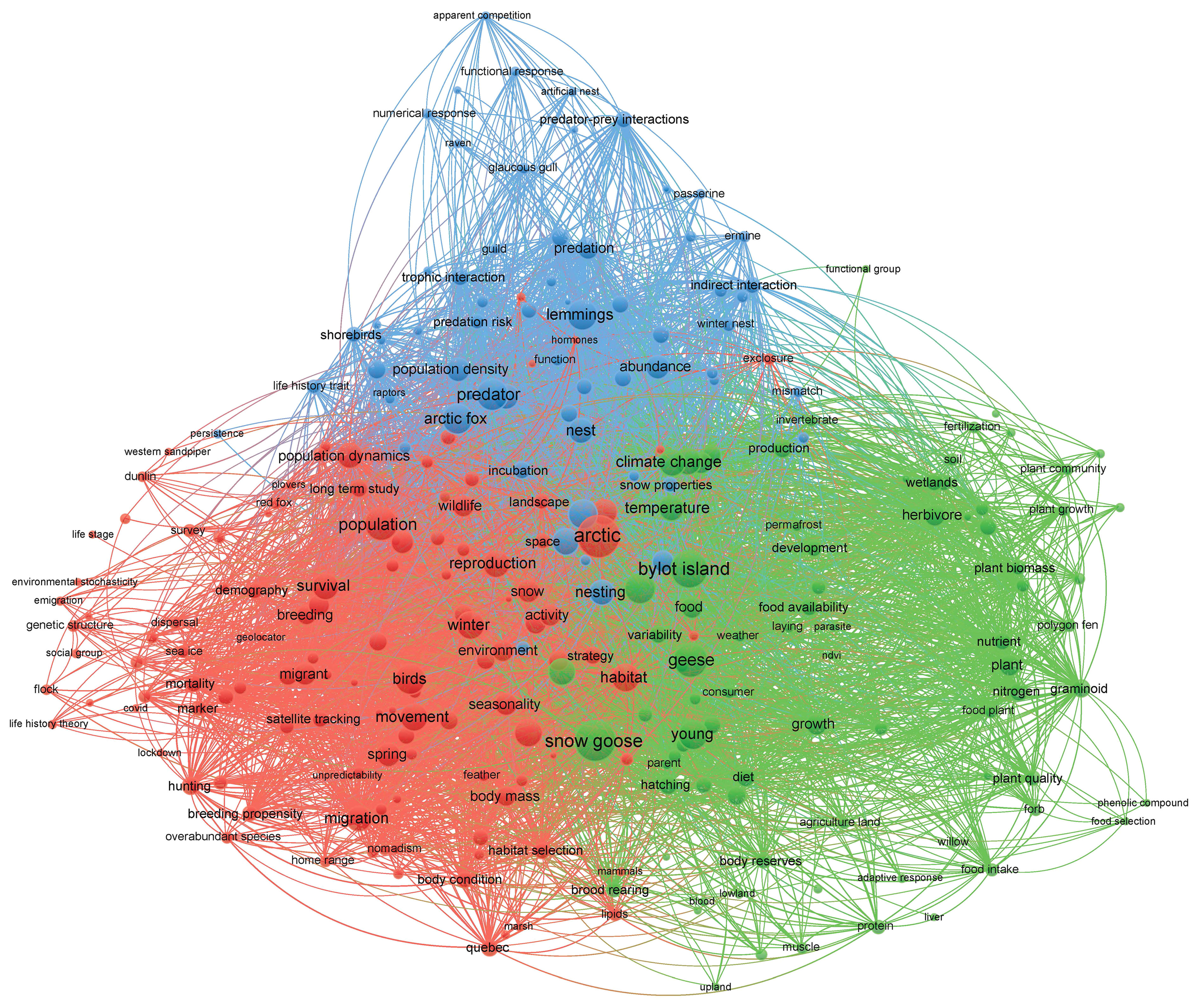
Figure 4 Bibliometric map illustrating the co-occurrence of terms in journal articles of the Bylot Island ecological studies. Circles size is proportional to the number of occurrences of a term and lines link terms with co-occurrences in publications (see methods in Supplementary Material for details). Colours illustrate clusters of terms with the highest number of links with each other.
5 Key scientific contributions
Plant-herbivore interactions have long been considered the dominant trophic interaction of the tundra food web based on empirical evidence and theory. Empirical evidence includes the strong impact of herbivores like colonial geese and migratory caribou on the tundra vegetation at some sites in the Arctic (Kerbes et al., 1990; Manseau et al., 1996; Jefferies et al., 2003). According to the exploitation ecosystem hypothesis developed 40 years ago (Oksanen et al., 1981), the low productivity of the tundra ecosystem did not support herbivore populations large enough to allow the persistence of predator populations of sufficient size to control herbivores (Oksanen and Oksanen, 2000). However, contrary to this theory, our work showed that predator-prey interactions were dominant in the Bylot Island food web. Predation was found to be the most important biotic factor affecting annual nesting success and productivity of snow geese (Gauthier et al., 2004; Morrissette et al., 2010; Juhasz et al., 2020) as well as the driving factor of lemming population fluctuations (Legagneux et al., 2012; Fauteux et al., 2015, Fauteux et al., 2016). Modelling work further suggested that seasonality was an essential component of lemming population dynamics and that their population cycles were not driven by a single predator but required a suite of predators, each with different life history characteristics (Hutchison et al., 2020; Bergeron, 2022). Lemming population fluctuations have a strong effect on numerous predators that depend upon them for their reproduction and survival (Therrien et al., 2014; Beardsell et al., 2016; Chevallier et al., 2020).
We also demonstrated that predator-prey interactions affect the whole tundra food web through indirect effects. When lemming populations crash, Arctic foxes show an immediate behavioral response and increase their predatory activity on bird nests (Bêty et al., 2002; Lecomte et al., 2008; Beardsell et al., 2022). We showed that predation level on bird nests is the outcome of a complex interaction between temporal fluctuations in lemming populations and spatial variation due to the presence of a large goose colony because both of these factors affect the reproduction, hunting behavior and spatial distribution of foxes (Giroux et al., 2012; Chevallier et al., 2020; Beardsell et al., 2022). Consequently, predation risk for shorebird nests increases when lemming abundance decreases as well as inside the goose colony (Lamarre et al., 2017; Dulude-de Broin et al., 2023), which may ultimately affect the persistence of local shorebird populations (Beardsell et al., 2023). Moreover, we provided evidence that the use of safer nesting habitat by some shorebird species may contribute to maintaining viable breeding populations over a broader distribution range (Léandri-Breton and Bêty, 2020). Overall, our results showed that predator-mediated effects can have significant consequences on the probability of occurrence of nesting bird species belonging to different trophic levels and guilds (Duchesne et al., 2021). Uncovering these subtle and complex interactions would not have been possible without both the insights and data provided by long-term monitoring.
The spatial flow of resources among ecosystems can affect food web dynamics of the receiving ecosystem, especially when the difference in productivity between ecosystems is high (Polis and Hurd, 1996; Polis et al., 1996). Our studies contributed to establish the prominence of allochthonous inputs from other ecosystems in shaping the food web of low productivity systems such as the Arctic tundra (Gauthier et al., 2011). For instance, input from the marine ecosystem due to local or regional animal movements help support Arctic fox and snowy owl populations (Therrien et al., 2011; Tarroux et al., 2012; Lai et al., 2017; Robillard et al., 2018). Another example is the food subsidy obtained by wintering geese in agriculture land that contributes to high goose populations and thus high prey abundance for foxes (Giroux et al., 2012). More recently, we developed the concept of community migration networks to characterize the structure of spatial connections created by migratory species, which dominate the Arctic food web (see Figure 1), as they winter in numerous ecoregions across the globe (Moisan et al., 2023). This new framework, rooted into the meta-ecosystem concept (Gounand et al., 2018), can be used to assess the seasonal transport of energy, contaminants, and diseases across ecosystems. It should also allow us to better predict how events occurring in distant areas can have cascading effects on trophic interactions in other ecosystems, and especially low productivity ones, through animal migration.
Like elsewhere in the Arctic, the Bylot Island ecosystem has experienced a strong warming trend over the course of our study (ranging from 0.8 to 1.2°C per decade depending of the season, except in mid-winter; Gauthier et al., 2011). Contrary to some other studies (e.g. Høye et al., 2007; Post et al., 2009), an analysis conducted 10 years ago did not reveal any change in the phenology, abundance or productivity of vertebrate species monitored on Bylot Island (Gauthier et al., 2013), a result that still largely holds today. The only exception is the recent increase in breeding cackling geese (Branta hutchinsii; J. Bêty, personal observations). This probably results from the large increase in the continental population associated with changes in land use in winter, and may reflect a possible northward range extension favored by warming. Our results thus suggest some resistance of the High Arctic tundra food web to the warming experienced so far (see also Hobbie et al., 2017; Schmidt et al., 2023). This may come to a surprise considering that we documented several ways by which climate warming may negatively affect Arctic vertebrates, such as through a trophic mismatch for geese (Doiron et al., 2015) or snow hardening for lemmings in winter (Poirier et al., 2021). This apparent paradox may be due to a lagged response of the food web or a non-linear response as species abundance may exhibit changes only when a certain threshold is reached. When this occurs, changes can occur abruptly, sometimes leading to catastrophic shifts (Scheffer et al., 2001). It is also possible that some studies that reported rapid changes in response to climate warming may have relied on time series too short (e.g. <10 years; see Schmidt et al., 2023) and were confounded by short-term effects of multiannual autocorrelation pattern in weather.
Ecosystem structure cannot be understood without a historical perspective. Red foxes (Vulpes vulpes), a species that negatively impacts Arctic foxes in many parts of the Arctic (Elmhagen et al., 2017), arrived on Bylot Island around 1950 (MacPherson, 1969). We had a strong interest in understanding this recent addition to our studied food web. We first analyzed genetic material to demonstrate that the twentieth century expansion of red foxes in the Canadian Arctic involved dispersers from nearby populations rather than European foxes introduced to North America (Berteaux et al., 2015). Red foxes on Bylot Island thus do not constitute an invading exotic species. Second, we analyzed Canadian fur harvest data from 1926–1950 (Gallant et al., 2020) to disentangle the relative influences of climate change and anthropogenic food subsidies in driving the red fox expansion. We demonstrated a strong effect of food subsidies and a marginal influence of climate. This case study shows how historical ecology can help interpret current monitoring by testing hypotheses about the relative influences of global ecological drivers.
Local and traditional indigenous knowledge can help cataloguing biodiversity, interpreting monitoring data, or building scientific hypotheses (Copete et al., 2023). However, much work is still needed to articulate and co-apply scientific and traditional knowledge. We worked with Inuit Elders and hunters from Mittimatalik, located near Bylot Island, to pair Indigenous knowledge with science-based knowledge related to Arctic fox and snow goose ecology (Gagnon and Berteaux, 2009). We showed that Indigenous knowledge can be of tremendous value to ecological monitoring (including high-tech monitoring) when it brings information at spatial and temporal scales not covered by scientific protocols. Equitably embracing multiple knowledge perspectives is not easy to achieve and brings epistemological challenges, but it is vital to the implementation of international agreements such as the Intergovernmental Science-Policy Platform on Biodiversity and Ecosystem Services (IPBES) and the Convention on Biological Diversity (CBD) (Tengö et al., 2017). This may explain why Gagnon and Berteaux (2009) became one of our most cited papers (Supplementary Table S1).
6 Scientific impact of ecological studies on Bylot Island
We evaluated the scientific impact of our long-term ecological studies using citation metrics for our journal articles (see methodological details in Supplementary Material). Our articles generated 7,740 citations (5,884 excluding autocitations) for a mean of 32.5 per publication (24.7 excluding autocitations) in the Web of Science (as of 24 August 2023; n = 238 peer-reviewed journal articles). Citations increased steadily over time and exceeded 600 annually recently (Figure 3). It is noteworthy that 4,316 publications cited our articles (4,083 excluding autocitations), which indicates that our work is cited by many authors. A more detailed analysis revealed that many of our publications are influential when compared to similar publications. We found that 65% of our articles (n = 238) had a number of citations above the median number of citations of articles in the same year, subject area and publication type, and 12% were above the 90% percentile (Supplementary Table S1). The latter category includes both articles where data from Bylot Island contributed to an international or national research published in high-ranking journals (e.g., McKinnon et al., 2010; Bulla et al., 2016; Berner et al., 2020; Davidson et al., 2020) as well as articles based on studies entirely conducted on Bylot Island (e.g., Bêty et al., 2002, Bêty et al., 2003; Gauthier et al., 2013; Doiron et al., 2015; Fauteux et al., 2015; Soininen et al., 2015).
As the Bylot Island long-term program gained momentum and international recognition due to its increasing contribution to scientific knowledge, our team members were increasingly invited to participate to international projects or assessments of the state and trends of the Arctic biodiversity. Articles falling into those categories (CIP and CIA, Figure 3) were absent during the first 15 years of the project but accounted for 19% (n = 190) of those published after 2005. During the International Polar Year (2007-2008), the Bylot Island team led the international project ArcticWOLVES (Arctic Wildlife Observatories Linking Vulnerable EcoSystems; Gauthier and Berteaux, 2011). The approach used on Bylot Island was exported to several other sites across the circumarctic through collaborations with researchers from 9 other countries over 3 years to better understand the functioning of the Arctic food web. This project alone generated over 150 peer-reviewed articles across the circumarctic region during the period 2007-2016. A key legacy of the ArcticWOLVES project was a much-needed spatial replication of food web studies similar to the one conducted on Bylot Island (see below for details).
More than 60 publications (including both peer-reviewed articles and reports) from the Bylot Island ecological studies were collectively cited over 100 times by policy documents between 1996 and 2023 (94% of citations since 2010; Source: Overton database, https://www.overton.io/). These publications include Gauthier et al. (2013) with 15 citations, Gauthier et al. (2005) with 11, Bêty et al. (2002) and Dickey et al. (2008) with 10, Legagneux et al. (2012) with 8, and Giroux et al. (2012) with 5. Policy documents citing our work come from a large diversity of organizations including the United Nations Environment Programme, the Intergovernmental Science-Policy Platform on Biodiversity and Ecosystem Services, the Intergovernmental Panel on Climate Change, the Arctic Council of Nordic countries as well as several national governments (Canada, USA, Norway, Sweden, Iceland, Germany, Poland, United Kingdom) and provincial or state governments (Alaska, California, Quebec, Nunavut). Collectively, we believe that these citation metrics illustrate the notable scientific and societal contributions of our long-term studies, a strong indicator of its success.
7 Ingredients of our success
It is important to highlight features that contributed to the success of a long-term ecological monitoring program (Lindenmayer and Likens, 2018). Here, we present 12 ingredients that contributed to the longevity of ecological studies on Bylot Island and their success.
1. Our program is a bottom-up initiative that emerged from the growing commitment of a small number of researchers with a diversity of expertise and interests. It started small and gradually expanded, especially during the first 15 years, as more food web components were added and new researchers joined the team (Figure 2). In doing so, we capitalized on strong personal motivation, and avoided much bureaucracy, which often characterizes “grand monitoring schemes” implemented all at once (i.e. the typical top-down approach). The bottom-up approach also provides increased flexibility in presence of unexpected constraints, such as those imposed by the Covid19 pandemic. In turn, researchers benefitted from the notoriety of the program to advance their career, a non-negligible benefit.
2. Since the beginning, our studies are articulated around clear objectives that are a mixture of fundamental science and more management-oriented questions. A secondary benefit of having goals originating from both basic and applied science is that it increases potential sources of funding for the program from agencies with different mandates. This has remained a hallmark of the project up to now.
3. The long-term monitoring was directed by a small number of dedicated university researchers under the leadership of the first author for more than 30 years. Good administration and overlap with new researchers who joined the team in recent years was important to ensure a smooth transition during the current transfer of leadership. The efficient transfer of ideas and knowledge among researchers and the collaborative efforts at securing funds for the project were also critical to build a common scientific vision, resolve practical difficulties, and ensure the year-after-year continuation of monitoring. Early-career researchers also received immediate benefits through graduate student cosupervisions and copublications.
4. Most of the research was conducted by graduate students and postdoctoral fellows under the supervision of the main researchers. Despite some challenges (see next section), the energy and dedication of graduate students injected a great dose of vitality to the program and contributed to the nurturing of the next generation of researchers. The continued renewal of students brings new ideas and sometimes improved ways of doing things. The subsequent recruitment of some of these students in the core team of researchers also helped expand the program and ensured its sustainability.
5. We need to be ready to accept surprises that challenge our previous understanding of the system and build from these surprises (Lindenmayer et al., 2010). The reorientation of our program from a primary focus on plant-herbivore to predator-prey interactions after the first decade emerged from initial field observations. The conceptual model that arose from this reorientation (Figure 1) helped us to adjust parameters monitored and sampling design to be sure to collect the information required to answer our questions and test our hypotheses of interest (Ims and Yoccoz, 2017). In retrospect, this conceptual model largely set the course for the success of our program.
6. A good knowledge of the study system and of the natural history of the species composing the food web is vital. This avoids gross misinterpretation of the data and may lead to unexpected field observations (e.g., Therrien et al., 2011; Chevallier et al., 2016; see also #5). We implemented the systematic collection of incidental wildlife observations during routine field work. This low-cost activity can be a highly valuable source of information and can provide, over the long-term, useful data to infer the relative abundance and change in phenology of many species. Our project provides several examples of that (e.g., Fauteux et al., 2018; Bolduc et al., 2023).
7. Acquisition of sound ecological knowledge can only be achieved through extensive presence in the field despite the high time and monetary costs involved. This has been mandatory in our program not only for students who are doing most of the field work but also for the main researchers, who need this experience as well. Although remote sensing (e.g., through field cameras or satellites) or automated data acquisition systems were useful additions (e.g., Lai et al., 2017; Kalhor et al., 2021), they cannot replace presence of investigators in the field. Besides, the presence of students and researchers at the field station together helped to forge a sense of belonging within the team, created emulation and was a formidable incubator of new ideas during discussions after long days spent in the field.
8.When collecting long-term data, it is vital to use quantitative, consistent, and repeatable methods adapted to the field site and stick to them, an approach that we adopted right from the start. All our field protocols are detailed in an in-house field manual, which expanded over the years as new components were added. This manual was essential to train new students over the years and ensured they had a resource to refer to when questions arose in the field. In a few situations where a change in methods was warranted, we made sure that there was a period of overlap between the two methods to validate them and maintain data continuity. Site comparison at the international level, such as the one achieved during the International Polar Year, can only be accomplished if protocols are harmonized and comparable (Berteaux et al., 2017). Several of our field protocols are included in technical manuals published online to the benefit of all (Cadieux et al., 2015, Cadieux et al., 2019; Lai and Berteaux, 2019).
9. Our project has combined several methodological approaches such as intensive short-term studies, long-term monitoring, experimental manipulations, and modelling. We believe that the combination of these approaches is highly desirable for research and training, and has considerably improved our understanding of the functioning of the food web.
10. Long-term projects are prone to generate a large amount of data and thus sound database management is of the utmost importance. This is particularly challenging with ecological data given the great diversity of data types collected, which comes primarily from observations by field workers and not recordings by automated sensors like temperature probes. This was initially a weakness of our program, primarily due to lack of sufficient funds, but the issue was progressively addressed and most data are now entered in relational databases. These databases are published on line in the collection NordicanaD maintained by the Centre d’études nordiques, Université Laval (https://nordicana.cen.ulaval.ca/) or on other global archives such as Movebank (https://www.movebank.org/cms/movebank-main). Nonetheless, regularly updating datasets remains a constant challenge.
11. It is important to consider multiple spatial scales and adjust them to questions or species of interest. For example, comparing animal behavior or distribution inside vs outside the goose colony revealed very important spatial patterns on Bylot Island (Giroux et al., 2012; Lamarre et al., 2017; Duchesne et al., 2021). The “one size fits all” should be avoided. For instance, small herbivores need to be monitored at a much smaller spatial scale (e.g., trapping grids of ca 10 ha) compared to mobile predators (e.g., foxes, at the scale of ca 500 km2) or migratory birds where thousands of kilometers need to be considered when examining connections with wintering areas (Moisan et al., 2023). Expansion of the spatial scale of our program over time allowed us to address increasingly complex questions at the scale of the whole food web.
12. Building and maintaining harmonious relations with the various stakeholders who have a vested interest in the research, and hold some power to decide who can use the study site and how, is also a key to the success of a long-term monitoring. In our case, partnerships with government agencies (primarily Environment and Climate Change Canada and Parks Canada) and the local Inuit community were established and maintained throughout the project.
It is interesting to note that many of these ingredients have been identified as key features of an effective ecological monitoring program by previous authors (Ims and Yoccoz, 2017; Lindenmayer and Likens, 2018).
8 Challenges of the program
We have identified six main challenges encountered during our long-term monitoring of the Bylot Island food web.
1. The lack of dedicated funding for long-term monitoring programs such as ours is a common problem (Lindenmayer, 2018) and we had to secure funding from multiple sources to maintain it. Most of our funding is short-term, which requires frequent grant renewal, a time-consuming task. One of the most critical components of Arctic field research is the helicopter and fixed-wing aircraft support that needs to be secured annually from a government agency in Canada (Polar Continental Shelf Program). Funding of this agency within the government is vulnerable to shifting political priorities and is thus periodically a source of concerns. The lack of stable, long-term funding dedicated specifically to monitoring may also lead to the termination of some time series in the long run although we have so far been able to avoid it. A related issue is that monitoring is generally not considered “rocket-science” by grant evaluation committees and thus the word “monitoring” should be avoided in many grant applications. On the one hand, this forces us to focus our research proposals on specific questions and hypotheses that can be addressed in the short-term, which is not a bad thing in itself. Nonetheless, this contributes to devaluating monitoring activities as it fails to recognize the enormous contribution of long-term data in answering these questions.
2. The long-term nature of the research does not fit well with the length of graduate student theses (typically 2-4 years). Although each student needs to collect data to answer the specific questions of his project, it is also important that each of them contributes to the collection of a core set of data (e.g., annual animal abundance) for the good of the long-term project. In some situations, such as when time available to do field work is limited, these dual objectives may enter in conflict and create tensions. Maintaining a good team spirit in the group and making sure that everybody understands the value of long-term data, including for their own project, helps to alleviate these problems. It is also important that experienced students returning to the field site take time to properly train new students to ensure that protocols used to collect long-term data are correctly applied. On a longer time-scale (20-30 years), interests and expertise can change between successive generations of researchers, potentially preventing some time series to expand beyond this time scale. It is thus important to have procedures in place that allow smooth transfer of expertise and datasets across generations of researchers to prevent the loss of important information including informal knowledge.
3. Another common problem of long-term studies is the lack of replication because it is often conducted at a single site (Lindenmayer and Likens, 2018), as in the case of the Bylot Island program. Therefore, we can never exclude the possibility that some of our results are due to peculiarities of the site, such as presence of a large goose colony or the coastal nature of the site in our case, which could cast some doubts on their generality. Fortunately, the large-scale project ArcticWOLVES funded during the International Polar Year (Gauthier and Berteaux, 2011) allowed us to replicate some of the food web studies conducted on Bylot Island at other circumpolar sites. A large-scale experiment showed the pervasive role of predation in promoting long-term migration (McKinnon et al., 2010). A comparative analysis also showed that the dominance of the predator-prey interaction in structuring the tundra food web of Bylot Island (Legagneux et al., 2012) was a general feature across the circumarctic tundra and not unique to our study site (Legagneux et al., 2014). It also revealed that herbivore body mass affected the strength of predator-prey interactions, with large herbivores, which are absent on Bylot Island, largely escaping predation. This illustrates well the power of long-term studies conducted at a single site in combination with short-term replications at other sites to confirm generality.
4. A related issue is the question of statistical design used to collect field data, which was not always ideal on Bylot Island. As examples, we can cite the lack of replication of our predator exclusion experiment (Fauteux et al., 2016) or the uneven spatial distribution of transects to assess prey abundance and distribution (Lamarre et al., 2017). Resources available and logistic constraints, such as the difficulty to reach sampling sites at the right time because people have to walk or wait for helicopter availability, are huge challenges when working at a remote Arctic site. In situations where true randomization was not possible, we strived to minimize potential biases using various approaches, such as a Before-After Control-Impact design for manipulations (Smith, 2013), selecting sites based on previous knowledge of the area to increase representativeness or repeat sampling over time. Whenever possible, we improved sampling design over time but made sure that it did not compromise the continuity of long time series.
5. In some systems, human-caused habitat disturbance can profoundly change cause-effect relationships in the studied system, or even prevent the continuation of experimental or observational protocols (Lindenmayer and Likens, 2018). Luckily, Bylot Island is a protected area (i.e. a national park) where major human disturbances have so far been avoided although some migratory species can be exposed to changes occurring on their wintering ground (e.g., Gauthier et al., 2005). We also strived to minimize researcher-induced disturbance and to test for its possible effect (e.g. Bêty and Gauthier, 2001). However, our monitoring program is not immune to other forms of disturbances such as factors preventing access to the site, which occurred for two years during the Covid19 pandemic and created unfortunate gaps in the data.
6.A related issue that can also affect the longevity and quality of long-term monitoring programs is the shifting priorities of stakeholders and local communities. It is especially relevant for our program because we are in a native territory and Inuit have to approve research permits. Increasing awareness of local communities to the importance of long-term environmental monitoring projects and of their participation in those projects is vital for their success. Developing and maintaining partnerships with local communities come with its own set of challenges, including distance, cultural differences, the need for trust-building, limited capacity in the communities and fatigue of local representatives when too many researchers are present in an area.
9 Conclusion
Robust monitoring is essential to provide sound baseline to detect future changes and to understand the mechanisms involved (Ims and Yoccoz, 2017). We believe that the Bylot Island ecological monitoring program provides a rare example of that in the Arctic, a region at the forefront of environmental changes due to rapid climate warming (Rantanen et al., 2022) but where field studies present unique challenges. We recognize that our program was not originally designed to address the consequences of climate change per se on Arctic ecosystem, but rather to understand the functioning of the Arctic food web. If the program had been designed specifically to address this question, some parameters could have been monitored differently or more of them could have been measured (Christensen et al., 2013). Nonetheless, we argue that our detailed studies oriented to understand mechanisms at work combined with the sustained measurement of several key components of the biota and of local climate conditions provide the essential ingredients required to detect and attribute most climate-driven changes occurring in this ecosystem. Moreover, integrating monitoring data and climate projections in food web models could help us better anticipate future change in this ecosystem, an approach that we recently initiated (Hutchison et al., 2020). We also believe that, as our program matured, its relevance largely exceeded the Bylot Island ecosystem and helped to shape broad ideas in ecology (e.g. McKinnon et al., 2010; Legagneux et al., 2014; Moisan et al., 2023).
Although the Bylot Island program has been so far highly successful, especially in terms of scientific impact and longevity, it is useful to project in the future and ask some questions. For instance, how long our ecological monitoring will remain relevant? How long should the program last? Thirty years may seem long because this is the average length of an academic career. It could be considered enough by many to understand key ecosystem processes and cover the range of natural variability. It could even be argued that 30 years provide a representative sample of periodic phenomena such as the 3-4-year lemming cycle, which is a major source of variability in the tundra ecosystem (Gauthier et al., 2024). On the other hand, 30 years remain a short period with respect to other ecological processes, such as the normal turnover of species that every ecosystem experiences. The same applies when trying to detect changes in ecosystem processes that may exhibit transient dynamics in response to environmental forcing (Hastings et al., 2018; Gauthier et al., 2024), or to determine whether the system has reached a tipping point leading to a state shift (Saulnier-Talbot et al., 2023). This is especially relevant in High Arctic systems like ours that have so far shown relatively little change in their biota despite ongoing warming (Gauthier et al., 2013; Schmidt et al., 2023).
Our Inuit partners often ask us “How long will you work here?”. Our typical answer is usually “We do not know”. Documenting, understanding and predicting the impacts of climate change on Arctic biodiversity and ecosystem functions are difficult tasks and it is unrealistic to think that these issues can be fully addressed over a fixed timeframe. Therefore, a more appropriate answer to this question could be “As long as we can”. Nonetheless, long-term ecological monitoring will always remain vulnerable to shifting scientific and political priorities, changing logistical constraints, and turnover within principal investigators. Regardless, our team will always remain committed to pursue the highest scientific standards possible in their ecological studies.
Author contributions
GG: Conceptualization, Data curation, Funding acquisition, Project administration, Writing – original draft, Writing – review & editing. DB: Conceptualization, Funding acquisition, Writing – original draft, Writing – review & editing. JB: Conceptualization, Funding acquisition, Writing – review & editing. PL: Conceptualization, Funding acquisition, Writing – review & editing. DF: Conceptualization, Writing – review & editing. DG: Conceptualization, Writing – review & editing. MC: Data curation, Writing – review & editing.
Funding
The author(s) declare that financial support was received for the research, authorship, and/or publication of this article. The numerous agencies that funded our ecological monitoring program since the beginning are listed in Supplementary Table S2.
Acknowledgments
We thank Philippe Boisvert for conducting the bibliometric analysis of our publications and all the graduate students and field assistants who have worked at the Bylot Island field station over the years. We also acknowledge Parks Canada and the Mittimatalik Hunter and Trapper Organization for allowing us to work on Bylot Island. Finally, we thank Rolf A. Ims and Justin Bastow for their constructive comments on our manuscript.
Conflict of interest
The authors declare that the research was conducted in the absence of any commercial or financial relationships that could be construed as a potential conflict of interest.
Publisher’s note
All claims expressed in this article are solely those of the authors and do not necessarily represent those of their affiliated organizations, or those of the publisher, the editors and the reviewers. Any product that may be evaluated in this article, or claim that may be made by its manufacturer, is not guaranteed or endorsed by the publisher.
Supplementary material
The Supplementary Material for this article can be found online at: https://www.frontiersin.org/articles/10.3389/fevo.2024.1359745/full#supplementary-material
References
Ankney C. D. (1996). An embarrassment of riches: Too many geese. J. Wildlife Manage. 60, 217–223. doi: 10.2307/3802219
Aronsson M., Heiðmarsson S., Jóhannesdóttir H., Barry T., Braa J., Burns C. T., et al. (2021). State of the arctic terrestrial biodiversity report (Akureyri, Iceland: Conservation of Arctic Flora and Fauna International Secretariat).
Barr S., Lüdecke C. (2010). The history of the international polar years (IPYs) (Heidelberg: Springer).
Beardsell A., Berteaux D., Dulude-De Broin F., Gauthier G., Clermont J., Gravel D., et al. (2023). Predator-mediated interactions through changes in predator home range size can lead to local prey exclusion. Proc. R. Soc. B 290, 20231154. doi: 10.1098/rspb.2023.1154
Beardsell A., Gauthier G., Fortier D., Therrien J.-F., Bêty J. (2017). Vulnerability to geomorphological hazards of an Arctic cliff-nesting raptor, the rough-legged hawk. Arctic Sci. 3, 203–219. doi: 10.1139/as-2016-0025
Beardsell A., Gauthier G., Therrien J. F., Bêty J. (2016). Nest site characteristics, patterns of nest reuse, and reproductive output in an arctic-nesting raptor, the rough-legged hawk. Auk 133, 718–732. doi: 10.1642/AUK-16-54.1
Beardsell A., Gravel D., Clermont J., Berteaux D., Gauthier G., Bêty J. (2022). A mechanistic model of functional response provides new insights into indirect interactions among arctic tundra prey. Ecology 103, e3734. doi: 10.1002/ecy.3734
Bergeron G. (2022). Approche simplifiée par états et transitions saisonniers pour modéliser un système prédateurs-proies complexe de l’Arctique. dépt. biologie, Université de Sherbrooke, Sherbrooke, QC.
Berner L. T., Massey R., Jantz P., Forbes B. C., Macias-Fauria M., Myers-Smith I., et al. (2020). Summer warming explains widespread but not uniform greening in the Arctic tundra biome. Nat. Commun. 11, 4621. doi: 10.1038/s41467-020-18479-5
Berteaux D., Gallant D., Sacks B. N., Statham M. J. (2015). Red foxes (Vulpes vulpes) at their expanding front in the Canadian Arctic have indigenous maternal ancestry. Polar Biol. 38, 913–917. doi: 10.1007/s00300-015-1647-6
Berteaux D., Thierry A. M., Alisauskas R., Angerbjörn A., Buchel E., Doronina L., et al. (2017). Harmonizing circumpolar monitoring of Arctic fox: benefits, opportunities, challenges and recommendations. Polar Res. 36, 2. doi: 10.1080/17518369.2017.1319602
Bêty J., Gauthier G. (2001). Effects of nest visits on predator activity and predation rate in a Greater Snow Goose colony. J. Field Ornithology 72, 573–586. doi: 10.1648/0273-8570-72.4.573
Bêty J., Gauthier G., Giroux J. F. (2003). Body condition, migration, and timing of reproduction in snow geese: A test of the condition-dependent model of optimal clutch size. Am. Nat. 162, 110–121. doi: 10.1086/375680
Bêty J., Gauthier G., Korpimaki E., Giroux J. F. (2002). Shared predators and indirect trophic interactions: lemming cycles and arctic-nesting geese. J. Anim. Ecol. 71, 88–98. doi: 10.1046/j.0021-8790.2001.00581.x
Bolduc E., Casajus N., Legagneux P., Mckinnon L., Gilchrist H. G., Leung M., et al. (2013). Terrestrial arthropod abundance and phenology in the Canadian Arctic: modelling resource availability for Arctic-nesting insectivorous birds. Can. Entomologist 145, 155–170. doi: 10.4039/tce.2013.4
Bolduc D., Fauteux D., Gagnon C. A., Gauthier G., Bêty J., Legagneux P. (2023). Testimonials to reconstruct past abundances of wildlife populations. Basic Appl. Ecol. 68, 23–34. doi: 10.1016/j.baae.2022.11.005
Bulla M., Valcu M., Dokter A. M., Dondua A. G., Kosztolányi A., Rutten A. L., et al. (2016). Unexpected diversity in socially synchronized rhythms of shorebirds. Nature 540, 109–113. doi: 10.1038/nature20563
Cadieux M. C., Fauteux D., Gauthier G. (2015). Technical manual for sampling small mammals in the Arctic − version 1 (Québec: Centre d’études nordiques, Université Laval).
Cadieux M. C., Gauthier G., Therrien J. F., Seyer Y., Beardsell A. (2019). Technical manual for monitoring avian predators of the Arctic tundra − Version 1 (Québec: Centre d’études nordiques, Université Laval).
CEN (2022). Climate station data from Bylot Island in Nunavut, Canada, v. 1.12.0 (1992-2022). Nordicana D2. doi: 10.5885/45039SL-EE76C1BDAADC4890
Chevallier C., Gauthier G., Lai S., Berteaux D. (2020). Pulsed food resources affect reproduction but not adult apparent survival in arctic foxes. Oecologia 193, 557–569. doi: 10.1007/s00442-020-04696-8
Chevallier C., Lai S., Berteaux D. (2016). Predation of Arctic Fox (Vulpes lagopus) Pups by Common Ravens (Corvus corax). Polar Biol. 39, 1335–1341. doi: 10.1007/s00300-015-1843-4
Christensen T., Payne J., Doyle M., Ibarguchi G., Taylor J., Schmidt N. M., et al. (2013). The arctic terrestrial biodiversity monitoring plan (Akureyri, Iceland: Conservation of Arctic Flora and Fauna International Secretariat).
Copete J. C., Kik A., Novotny V., Cámara-Leret R. (2023). The importance of Indigenous and local people for cataloging biodiversity. Trends Ecol. Evol. 38, 1112–1114. doi: 10.1016/j.tree.2023.08.017
Davidson S. C., Bohrer G., Gurarie E., LaPoint S., Mahoney P. J., Boelman N. T., et al. (2020). Ecological insights from three decades of animal movement tracking across a changing Arctic. Science 370, 712–715. doi: 10.1126/science.abb7080
Deschamps L., Maire V., Chen L., Fortier D., Gauthier G., Morneault A., et al. (2023). Increased nutrient availability speeds up permafrost development, while goose grazing slows it down in a Canadian High Arctic wetland. J. Ecol. 111, 449–463. doi: 10.1111/1365-2745.14037
Dickey M. H., Gauthier G., Cadieux M. C. (2008). Climatic effects on the breeding phenology and reproductive success of an arctic-nesting goose species. Global Change Biol. 14, 1973–1985. doi: 10.1111/j.1365-2486.2008.01622.x
Doiron M., Gauthier G., Levesque E. (2015). Trophic mismatch and its effects on the growth of young in an Arctic herbivore. Global Change Biol. 21, 4364–4376. doi: 10.1111/gcb.13057
Domine F., Belke-Brea M., Sarrazin D., Arnaud L., Barrere M., Poirier M. (2018a). Soil moisture, wind speed and depth hoar formation in the Arctic snowpack. J. Glaciology 64, 990–1002. doi: 10.1017/jog.2018.89
Domine F., Gauthier G., Vionnet V., Fauteux D., Dumont M., Barrere M. (2018b). Snow physical properties may be a significant determinant of lemming population dynamics in the high Arctic. Arctic Sci. 4, 813–826. doi: 10.1139/as-2018-0008
Duchesne É., Lamarre J. F., Gauthier G., Berteaux D., Gravel D., Bêty J. (2021). Variable strength of predator-mediated effects on species occurrence in an arctic terrestrial vertebrate community. Ecography 44, 1236–1248. doi: 10.1111/ecog.05760
Dulude-de Broin F., Clermont J., Beardsell A., Ouellet L. P., Legagneux P., Bêty J., et al. (2023). Predator home range size mediates indirect interactions between prey species in an arctic vertebrate community. J. Anim. Ecol 92, 2373–2385. doi: 10.1111/1365-2656.14017
Elmhagen B., Berteaux D., Burgess R. M., Ehrich D., Gallant D., Henttonen H., et al. (2017). Homage to hersteinsson and macdonald: climate warming and resource subsidies cause red fox range expansion and arctic fox decline. Polar Res. 36, 3. doi: 10.1080/17518369.2017.1319109
Fauteux D., Gauthier G., Berteaux D. (2015). Seasonal demography of a cyclic lemming population in the Canadian Arctic. J. Anim. Ecol. 84, 1412–1422. doi: 10.1111/1365-2656.12385
Fauteux D., Gauthier G., Berteaux D. (2016). Top-down limitation of lemmings revealed by experimental reduction of predators. Ecology 97, 3231–3241. doi: 10.1002/ecy.1570
Fauteux D., Gauthier G., Mazerolle M. J., Coallier N., Bêty J., Berteaux D. (2018). Evaluation of invasive and non-invasive methods to monitor rodent abundance in the arctic. Ecosphere 9, 2124. doi: 10.1002/ecs2.2124
Ferguson S. H., Berteaux D., Gaston A. J., Higdon J. W., Lecomte N., Lunn N., et al. (2012). Time series data for Canadian arctic vertebrates: IPY contributions to science, management, and policy. Climatic Change 115, 235–258. doi: 10.1007/s10584-012-0476-7
Gagnon C. A., Berteaux D. (2009). Integrating traditional ecological knowledge and ecological science: a question of scale. Ecol. Soc. 14, 19. doi: 10.5751/ES-02923-140219
Gallant D., Lecomte N., Berteaux D. (2020). Disentangling the relative influences of global drivers of change in biodiversity: A study of the twentieth-century red fox expansion into the Canadian Arctic. J. Anim. Ecol. 89, 565–576. doi: 10.1111/1365-2656.13090
Gaston A. J., Cyr M. A., O'Donovan K. (2017). A first count of Thick-billed Murres (Uria lomvia) and Black-legged Kittiwakes (Rissa tridactyla) breeding on Bylot Island. Can. Field-Naturalist 131, 69–74. doi: 10.22621/cfn.v131i1.1953
Gauthier G., Berteaux D. (2011). ArcticWOLVES: Arctic Wildlife Observatories Linking Vulnerable EcoSystems. Final synthesis report (Quebec: Université Laval, Centre d’études nordiques).
Gauthier G., Berteaux D., Bêty J., Tarroux A., Therrien J. F., Mckinnon L., et al. (2011). The tundra food web of Bylot Island in a changing climate and the role of exchanges between ecosystems. Ecoscience 18, 223–235. doi: 10.2980/18-3-3453
Gauthier G., Bêty J., Cadieux M. C., Legagneux P., Doiron M., Chevallier C., et al. (2013). Long-term monitoring at multiple trophic levels suggests heterogeneity in responses to climate change in the Canadian Arctic tundra. Philos. Trans. R. Soc. B 368, 20482. doi: 10.1098/rstb.2012.0482
Gauthier G., Bêty J., Giroux J. F., Rochefort L. (2004). Trophic interactions in a High Arctic snow goose colony. Integr. Comp. Biol. 44, 119–129. doi: 10.1093/icb/44.2.119
Gauthier G., Cadieux M.-C., Berteaux D., Bêty J., Fauteux D., Legagneux P., et al. (2023). Long-term study of the tundra food web at a hotspot of Arctic biodiversity, the Bylot Island Field Station. Arctic Sci. doi: 10.1139/as-2023-0029
Gauthier G., Ehrich D., Belke-Brea M., Domine F., Alisauskas R., Clark K., et al. (2024). Taking the beat of the Arctic: are lemming population cycles changing due to winter climate? Proc. R. Soc. B 291, 20232361. doi: 10.1098/rspb.2023.2361
Gauthier G., Giroux J. F., Reed A., Bechet A., Belanger L. (2005). Interactions between land use, habitat use, and population increase in greater snow geese: what are the consequences for natural wetlands? Global Change Biol. 11, 856–868. doi: 10.1111/j.1365-2486.2005.00944.x
Giroux M. A., Berteaux D., Lecomte N., Gauthier G., Szor G., Bêty J. (2012). Benefiting from a migratory prey: spatio-temporal patterns in allochthonous subsidization of an arctic predator. J. Anim. Ecol. 81, 533–542. doi: 10.1111/j.1365-2656.2011.01944.x
Godin E., Fortier D., Levesque E. (2016). Nonlinear thermal and moisture response of ice-wedge polygons to permafrost disturbance increases heterogeneity of high arctic wetland. Biogeosciences 13, 1439–1452. doi: 10.1088/1748-9326/9/10/105010
Gounand I., Harvey E., Little C. J., Altermatt F. (2018). Meta-ecosystems 2.0: rooting the theory into the field. Trends Ecol. Evol. 33, 36–46. doi: 10.1016/j.tree.2017.10.006
Hastings A., Abbott K. C., Cuddington K., Francis T., Gellner G., Lai Y.-C., et al. (2018). Transient phenomena in ecology. Science 361, eaat6412. doi: 10.1126/science.aat6412
Hobbie J. E., Shaver G. R., Rastetter E. B., Cherry J. E., Goetz S. J., Guay K. C., et al. (2017). Ecosystem responses to climate change at a low arctic and a high arctic long-term research site. Ambio 46, S160–S173. doi: 10.1007/s13280-016-0870-x
Høye T., Post E., Meltofte H., Schmidt N. M., Forchammer M. C. (2007). Rapid advancement of spring in the High Arctic. Curr. Biol. 17, R449–R451. doi: 10.1016/j.cub.2007.04.047
Hutchison C., Guichard F., Legagneux P., Gauthier G., Bêty J., Berteaux D., et al. (2020). Seasonal food webs with migrations: multi-season models reveal indirect species interactions in the Canadian Arctic tundra. Philos. Trans. R. Soc. A 378, 20190354. doi: 10.1098/rsta.2019.0354
Ims R. A., Jepsen J. U., Stien A., Yoccoz N. G. (2013). Science plan for COAT: climate-ecological observatory for arctic tundra (Tromsø, Norway: Fram Centre by the University of Tromsø).
Ims R. A., Yoccoz N. G. (2017). Ecosystem-based monitoring in the age of rapid climate change and new technologies. Curr. Opin. Environ. Sustainability 29, 170–176. doi: 10.1016/j.cosust.2018.01.003
Jefferies R. L., Rockwell R. F., Abraham K. F. (2003). The embarrassment of riches: agricultural food subsidies, high goose numbers, and loss of Arctic wetlands - a continuing saga. Environ. Rev. 11, 193–232. doi: 10.1139/A04-002
Juhasz C. C., Shipley B., Gauthier G., Berteaux D., Lecomte N. (2020). Direct and indirect effects of regional and local climatic factors on trophic interactions in the Arctic tundra. J. Anim. Ecol. 89, 704–715. doi: 10.1111/1365-2656.13104
Kalhor D., Poirier M., Pusenkova A., Maldague X., Gauthier G., Galstian T. (2021). A camera trap to reveal the obscure world of the arctic subnivean ecology. IEEE Sensors J. 21, 28025–28036. doi: 10.1109/JSEN.2021.3122203
Kerbes R. H., Kotanen P. M., Jefferies R. L. (1990). Destruction of wetland habitats by lesser snow geese: a keystone species on the west coast of Hudson Bay. J. Appl. Ecol. 27, 242–258. doi: 10.2307/2403582
Lai S., Berteaux D. (2019). Technical manual for monitoring foxes in the Arctic − Version 1 (Rimouski: Canada Research Chair on Northern Biodiversity, Université du Québec à Rimouski).
Lai S., Bêty J., Berteaux D. (2017). Movement tactics of a mobile predator in a meta-ecosystem with fluctuating resources: the arctic fox in the high arctic. Oikos 126, 937–947. doi: 10.1111/oik.03948
Lamarre J. F., Legagneux P., Gauthier G., Reed E. T., Bêty J. (2017). Predator-mediated negative effects of overabundant snow geese on arctic-nesting shorebirds. Ecosphere 8, 1788. doi: 10.1002/ecs2.1788
Léandri-Breton D. J., Bêty J. (2020). Vulnerability to predation may affect species distribution: plovers with broader arctic breeding range nest in safer habitat. Sci. Rep. 10, 5032. doi: 10.1038/s41598-020-61956-6
Lecomte N., Careau V., Gauthier G., Giroux J. F. (2008). Predator behaviour and predation risk in the heterogeneous Arctic environment. J. Anim. Ecol. 77, 439–447. doi: 10.1111/j.1365-2656.2008.01354.x
Legagneux P., Gauthier G., Berteaux D., Bêty J., Cadieux M. C., Bilodeau F., et al. (2012). Disentangling trophic relationships in a High Arctic tundra ecosystem through food web modeling. Ecology 93, 1707–1716. doi: 10.1890/11-1973.1
Legagneux P., Gauthier G., Lecomte N., Schmidt N. M., Reid D., Cadieux M. C., et al. (2014). Arctic ecosystem structure and functioning shaped by climate and herbivore body size. Nat. Climate Change 4, 379–383. doi: 10.1038/NCLIMATE2168
Lepage D., Gauthier G., Reed A. (1996). Breeding-site infidelity in greater snow geese: A consequence of constraints on laying date? Can. J. Zoology 74, 1866–1875. doi: 10.1139/z96-210
Lepage D., Nettleship D. N., Reed A. (1998). Birds of Bylot Island and adjacent Baffin Island, Northwest Territories, Canada 1979 to 1997. Arctic 51, 125–141. doi: 10.14430/arctic1054
Lindenmayer D. (2018). Why is long-term ecological research and monitoring so hard to do? (And what can be done about it). Aust. Zoologist 39, 576–580. doi: 10.7882/az.2017.018
Lindenmayer D. B., Likens G. E. (2018). Effective ecological monitoring (Clayton South: CSIRO Publishing).
Lindenmayer D. B., Likens G. E., Krebs C. J., Hobbs R. J. (2010). Improved probability of detection of ecological "surprises". Proc. Natl. Acad. Sci. 107, 21957–21962. doi: 10.1073/pnas.1015696107
MacPherson A. H. (1969). The dynamics of Canadian Arctic fox populations (Ottawa: Canadian Wildlife Service Report Series).
Mallory M. L., Gilchrist H. G., Janssen M., Major H. L., Merkel F., Provencher J. F., et al. (2018). Financial costs of conducting science in the Arctic: examples from seabird research. Arctic Sci. 4, 624–633. doi: 10.1139/as-2017-0019
Manseau M., Huot J., Crête M. (1996). Effects of summer grazing by caribou on composition and productivity of vegetation: Community and landscape level. J. Ecol. 84, 503–513. doi: 10.2307/2261473
McKinnon L., Smith P. A., Nol E., Martin J. L., Doyle F. I., Abraham K. F., et al. (2010). Lower predation risk for migratory birds at high latitudes. Science 327, 326–327. doi: 10.1126/science.1183010
Meltofte H. (2013). Arctic Biodiversity Assessment: Status and trend in Arctic biodiversity (Akureyri, Iceland: Conservation of Arctic Flora and Fauna International Secretariat).
Moisan L., Gravel D., Legagneux P., Gauthier G., Léandri-Breton D. J., Somveille M., et al. (2023). Scaling migrations to communities: An empirical case of migration network in the Arctic. Front. Ecol. Evol. 10. doi: 10.3389/fevo.2022.1077260
Morrissette M., Bêty J., Gauthier G., Reed A., Lefebvre J. (2010). Climate, trophic interactions, density dependence and carry-over effects on the population productivity of a migratory Arctic herbivorous bird. Oikos 119, 1181–1191. doi: 10.1111/j.1600-0706.2009.18079.x
Oksanen L., Fretwell D. S., Arruda J., Niemela P. (1981). Exploitation ecosystems in gradients of primary productivity. Am. Nat. 118, 240–261. doi: 10.1086/283817
Oksanen L., Oksanen T. (2000). The logic and realism of the hypothesis of exploitation ecosystems. Am. Nat. 155, 703–723. doi: 10.1086/303354
Poirier M., Fauteux D., Gauthier G., Domine F., Lamarre J. F. (2021). Snow hardness impacts intranivean locomotion of arctic small mammals. Ecosphere 12, e03835. doi: 10.1002/ecs2.3835
Polis G. A., Holt R. D., Menge B. A., Winemiller K. O. (1996). “Time, space, and life history: Influences on food webs,” in Food webs: integration of patterns and dynamics. Eds. Polis G. A., Winemiller K. O. (Chapman & Hall, New York, New York), 435–460.
Polis G. A., Hurd S. D. (1996). Linking marine and terrestrial food webs: allochthonous input from the ocean supports high secondary productivity on small islands and coastal land communities. Am. Nat. 147, 396–423.doi: 10.1086/285858
Post E., Forchhammer M. C., Bret-Harte M. S., Callaghan T. V., Christensen T. R., Elberling B., et al. (2009). Ecological dynamics across the Arctic associated with recent climate change. Science 325, 1355–1358. doi: 10.1126/science.1173113
Préskienis V., Laurion I., Bouchard F., Douglas P. M. J., Billett M. F., Fortier D., et al. (2021). Seasonal patterns in greenhouse gas emissions from lakes and ponds in a High Arctic polygonal landscape. Limnology Oceanography 66, S117–S141. doi: 10.1002/lno.11660
Rantanen M., Karpechko A. Y., Lipponen A., Nordling K., Hyvärinen O., Ruosteenoja K., et al. (2022). The Arctic has warmed nearly four times faster than the globe since 1979. Commun. Earth Environ. 3, 168. doi: 10.1038/s43247-022-00498-3
Reed A., Hughes R. J., Boyd H. (2002). Patterns of distribution and abundance of Greater Snow Geese on Bylot Island, Nunavut, Canada 1983-1998. Wildfowl 53, 53–65.
Rew L. J., McDougall K. L., Alexander J. M., Daehler C. C., Essl F., Haider S., et al. (2020). Moving up and over: redistribution of plants in alpine, Arctic, and Antarctic ecosystems under global change. Arctic Antarctic Alpine Res. 52, 651–665. doi: 10.1080/15230430.2020.1845919
Robillard A., Gauthier G., Therrien J. F., Bêty J. (2018). Wintering space use and site fidelity in a nomadic species, the snowy owl. J. Avian Biol. 49, e01707. doi: 10.1111/jav.01707
Saulnier-Talbot E., Duchesne E., Antoniades D., Arseneault D., Barnard C., Berteaux D., et al. (2023). State shifts and divergent sensitivities to climate warming across northern ecosystems. Res. Square. doi: 10.21203/rs.3.rs-3026284/v1
Scheffer M., Carpenter S., Foley J. A., Folke C., Walker B. (2001). Catastrophic shifts in ecosystems. Nature 413, 591–596. doi: 10.1038/35098000
Schmidt N. M., Kankaanpää T., Tiusanen M., Reneerkens J., Versluijs T. S. L., Hansen L. H., et al. (2023). Little directional change in the timing of Arctic spring phenology over the past 25 years. Curr. Biol. 33, 3244–3249. doi: 10.1016/j.cub.2023.06.038
Smith E. P. (2013). BACI design. Encyclopedia Environmetrics. doi: 10.1002/9780470057339.vab001.pub2
Soininen E. M., Gauthier G., Bilodeau F., Berteaux D., Gielly L., Taberlet P., et al. (2015). Highly overlapping winter diet in two sympatric lemming species revealed by DNA metabarcoding. PloS One 10, e0115335. doi: 10.1371/journal.pone.0115335
Tarroux A., Bêty J., Gauthier G., Berteaux D. (2012). The marine side of a terrestrial carnivore: intra-population variation in use of allochthonous resources by arctic foxes. PloS One 7, e42427. doi: 10.1371/journal.pone.0042427
Tengö M., Hill R., Malmer P., Raymond C. M., Spierenburg M., Danielsen F., et al. (2017). Weaving knowledge systems in IPBES, CBD and beyond—lessons learned for sustainability. Curr. Opin. Environ. Sustainability 26-27, 17–25. doi: 10.1016/j.cosust.2016.12.005
Therrien J. F., Gauthier G., Bêty J. (2011). An avian terrestrial predator of the arctic relies on the marine ecosystem during winter. J. Avian Biol. 42, 363–369. doi: 10.1111/j.1600-048X.2011.05330.x
Therrien J. F., Gauthier G., Korpimaki E., Bêty J. (2014). Predation pressure by avian predators suggests summer limitation of small-mammal populations in the Canadian Arctic. Ecology 95, 56–67. doi: 10.1890/13-0458.1
Tolvanen A., Eilu P., Juutinen A., Kangas K., Kivinen M., Markovaara-Koivisto M., et al. (2019). Mining in the Arctic environment - A review from ecological, socioeconomic and legal perspectives. J. Environ. Manage. 233, 832–844. doi: 10.1016/j.jenvman.2018.11.124
Walker D. A., Raynolds M. K., Daniels F. J. A., Einarsson E., Elvebakk A., Gould W. A., et al. (2005). The circumpolar vegetation map. J. Vegetation Sci. 16, 267–285. doi: 10.1111/j.1654-1103.2005.tb02365.x
Willig M. R., Bloch C. P., Ranta E. (2006). Latitudinal gradients of species richness: A test of the geographic area hypothesis at two ecological scales. Oikos 112, 163–173. doi: 10.1111/j.0030-1299.2006.14009.x
Keywords: arctic ecosystem, food web, climate change, long-term study, biodiversity, baseline information
Citation: Gauthier G, Berteaux D, Bêty J, Legagneux P, Fauteux D, Gravel D and Cadieux M-C (2024) Scientific contributions and lessons learned from 30 years of ecological monitoring of the Bylot Island tundra ecosystem. Front. Ecol. Evol. 12:1359745. doi: 10.3389/fevo.2024.1359745
Received: 21 December 2023; Accepted: 01 March 2024;
Published: 19 March 2024.
Edited by:
Dennis Murray, Trent University, CanadaReviewed by:
Rolf A. Ims, UiT The Arctic University of Norway, NorwayJustin Bastow, Eastern Washington University, United States
Copyright © 2024 Gauthier, Berteaux, Bêty, Legagneux, Fauteux, Gravel and Cadieux. This is an open-access article distributed under the terms of the Creative Commons Attribution License (CC BY). The use, distribution or reproduction in other forums is permitted, provided the original author(s) and the copyright owner(s) are credited and that the original publication in this journal is cited, in accordance with accepted academic practice. No use, distribution or reproduction is permitted which does not comply with these terms.
*Correspondence: Gilles Gauthier, gilles.gauthier@bio.ulaval.ca