Patterns of morphological diversification are influenced by dietary evolution in a highly species-rich lizard radiation
- 1Departamento de Biología, Doctorado en Ciencias Biológicas, Ecología de Zonas Áridas (EZA), Universidad de La Serena, La Serena, Chile
- 2Red de Investigadores en Herpetología-Bolivia, La Paz, Bolivia
- 3Unidad de Zoología – Instituto de Ecología, Universidad Mayor de San Andrés, La Paz, Bolivia
- 4MacroBiodiversity Lab, School of Biological Sciences, Queen’s University Belfast, Belfast, United Kingdom
- 5Instituto Multidisciplinario de Investigación y Posgrado, Universidad de La Serena, La Serena, Chile
The diversification of lineages is facilitated or constrained by the simultaneous evolution of multiple components of the phenotype that interact with each other during the course of speciation. When evolutionary radiations are adaptive, lineages proliferate via the emergence of multiple phenotypic optima that underlie diversification of species across multiple ecological niches. When radiations are non-adaptive, lineage proliferation unfolds constrained by similar (or nearly identical) correlations among traits that keep phenotypic and ecological diversity across newly emerging species within a single optimum. Nature offers very few opportunities where both types of diversification occur between closely related and highly diverse lineages. The Liolaemidae family of South American lizards offers unique such opportunities given two speciose lineages that have rapidly proliferated via adaptive (Liolaemus) and non-adaptive (Phymaturus) radiations. We analyze body shape in lizards in association with type of diet (herbivory, omnivory or carnivory). In these lizards, diet types have been suggested to be linked to body size. Our results confirm this hypothesis, with three body size optima tightly linked to all three diet types when radiation is both adaptive and non-adaptive. Diet reconstruction along their evolutionary history showed that the common ancestor of Liolaemidae was likely omnivorous, which is matched by ancestral reconstruction of body size. Phylogenetic PCA revealed that herbivorous species generally have more differentiated body shape than insectivores and omnivores. Herbivorous species have evolved larger heads, shorter hindlimbs and a small difference between forelimb and hindlimb length. In contrast, omnivores and insectivores have smaller heads and longer hindlimbs. Collectively, trophic niche plays an important role in defining body shape and size across species within lineages, and the patterns of trait–ecology correlations remain consistent when lineages have diversified via adaptive and non-adaptive radiation.
1 Introduction
The adaptive radiation of lineages is triggered when ecological opportunity – abundance in available niche space – emerges as a consequence of the formation of new environments, large-scale extinctions or the evolution of a key adaptive innovation that facilitates exploitation of niches that were previous inaccessible (Schluter, 2000). During adaptive radiation species diverge into ecologically distinct lineages that driven by natural selection, exploit their habitat in different ways (Pincheira-Donoso et al., 2018; Matsubayashi and Yamaguchi, 2020). In contrast, non-adaptive radiation is characterized by a species diversification with minimal ecological difference occupying similar niche space, and may be associated with variation in sexual selection across populations (Czekanski-Moir and Rundell, 2019). Although there are fewer studies on non-adaptive radiation, it seems to be more common than expected (Rundell and Price, 2009; Reaney et al., 2018; Czekanski-Moir and Rundell, 2019; Matsubayashi and Yamaguchi, 2020).
Adaptation to food is a major source of natural selection. Therefore, the availability and diversity of trophic resources can play a central role during adaptive divergence (Price et al., 2012; Burin et al., 2016; Ocampo et al., 2022), which is often associated with co-adaptation in morphological traits (Grant and Grant, 2003; Stokstad, 2004; Eloy de Amorim et al., 2017). Adaptive morphological changes linked to diet may have, in some cases, a greater effect than interspecific competition or predation (Jones et al., 2013). This functional association between the evolution of diet and morphological traits is expected to, therefore, influence patterns of morphological diversity within lineages, with convergent evolution in body plans (body size and shape) among species with similar diets, and divergent adaptations among species that exploit different resources. Many examples of convergence have been found across different animal groups, such as cichlid fish, anolid lizards and mammal species, mainly attributed to the link between niche availability and resource use (Muschick et al., 2012; Mahler et al., 2013; Mazel et al., 2017; Gearty et al., 2018).
The shape of the head, body, and limbs is strongly influenced by the type of diet, playing a significant role in feeding habits and food processing (Grant and Grant, 2002; Stokstad, 2004; O’Grady et al., 2005; Miles et al., 2007; Eloy de Amorim et al., 2017; Pincheira-Donoso, 2021). For example, cranial morphology and size in vertebrates mirror specific diet types given that head and skull are key for obtaining, processing and ingesting certain food items and thus, are subject to certain mechanical constraints depending on food type (Dollion et al., 2017). In the tropidurid lizard Microlophus thoracicus, ontogenetic changes in diet are coupled with changes in head shape. In this species adults are herbivores and exhibit proportionally wider and more robust heads, whereas insectivorous juveniles have narrower and slender heads (Toyama et al., 2018). Body size is also an important factor for diet since herbivores tend to be bigger in size than their non-herbivore sister taxa (Price et al., 2012), given that nutritional value of plant material is lower than animal-based material that high in protein. Consequently, herbivorous species compensate for a low nutritional value with greater body volume for digestion (Pough, 1973). On the other hand, insectivorous species tend to have small bodies and thin heads because they require more agility to capture insects for food (Szarski, 1962; Pough, 1973). In a similar environment, these traits tend to converge around an evolutionary optima value (O’Meara and Beaulieu, 2014).
Changes towards new evolutionary optima in functional morphology are driven by natural selection for a more efficient exploitation of new food resources, optimal foraging and efficient consumption time (Price et al., 2012). These evolutionary optima can be estimated using different Ornstein–Uhlenbeck (OU) models, which have the ability to better fit empirical data, as well as draw biological conclusions based on their parameter estimates (O’Meara and Beaulieu, 2014). The use of these models has increased the understanding of the ecological and evolutionary processes underlying species diversification as shown by various studies on different vertebrate groups (Lapiedra et al., 2013; Astudillo-Clavijo et al., 2015; Pincheira-Donoso et al., 2015; Gearty et al., 2018; Lapiedra et al., 2021). These methods are a good fit for highly diverse and broadly distributed groups. Here, we use this approach to explore how evolutionary changes in diet have driven distinct evolutionary optima of ecomorphological traits related to resource use in the lizard family Liolaemidae.
The Liolaemidae family is a group of South American lizards with great diversity (340 species into 3 genera) (Uetz et al., 2023), inhabiting a wide variety of environments across its range in the southern half of the continent (Pincheira-Donoso et al., 2008a; Pincheira-Donoso et al., 2008b; Pincheira-Donoso et al., 2009; Abdala and Quinteros, 2014; Roll et al., 2017; Esquerré et al., 2019). Species of the family are distributed from sea level to extreme high elevations ranging from 5000 to 5400 m (Aparicio and Ocampo, 2010; Pincheira-Donoso et al., 2013; Cerdeña et al., 2021). This family has two reproductive modes (oviparous, viviparous), and three diet types (insectivore, omnivore, herbivore) (Pincheira-Donoso et al., 2008b; Pincheira-Donoso and Tregenza, 2011; Pincheira-Donoso et al., 2013; Pincheira-Donoso et al., 2017; Zimin et al., 2022). All of these traits have been shown to contribute to the diversification of the family and promote the occupancy of novel niche space (Espinoza et al., 2004; Esquerré et al., 2019). Recent findings have unveiled the pivotal role of dietary evolution in the evolutionary radiation of the group, where evolutionary transitions from insectivory to herbivory, bridged by omnivory, have provided ecological opportunities for the rapid and successful diversification across a variety of climates (Ocampo et al., 2022). Just as in mammals (Price et al., 2012), herbivorous Liolaemus have evolved greater body sizes associated with other morphological changes (Pough, 1973; Cooper Jr. and Vitt, 2002). For example, O’Grady et al. (2005) compared body and gut size among 22 species of Liolaemus with different diets (herbivory, omnivory and insectivory) showing that herbivorous species have evolved bigger and longer digestive tract. We are yet to unravel, however, how changes in diet (source of ecological opportunity) influence changes and variation in functional ecomorphological traits and body size as a result of ecological release that triggered radiation of the group.
In Liolaemidae, the genus Phymaturus that is almost entirely composed of herbivorous species, it is believed to have diversified through non-adaptive radiation. Quite the reverse, the genus Liolaemus, which is species rich and harbors herbivorous, insectivorous and omnivorous species that commonly evolved through convergence, is believed to have diversified through adaptive radiation (Reaney et al., 2018). Therefore, in this study we hypothesize that in Liolaemidae evolutionary changes in diet type, imposed by novel selective pressures, have influenced changes in ecomorphology and body size adaptations across species in accord with the diversification of the family. We expect, traits related to the head, body, and limbs to show different evolutionary optima values across its evolutionary history under an OU process and in accordance with diet evolution. Lineages that transitioned to an herbivorous diet should have evolved a larger body, which supports longer intestines that facilitate the digestion of plant material (Clauss et al., 2013; Pincheira-Donoso, 2021). They should have also evolved optima for robust limbs as compared to omnivorous lineages. In addition, a thick skull optimum indicative of a stronger bite force should be prevalent (Herrel et al., 1999, Herrel et al., 2004). On the contrary, insectivorous lineages should have evolved towards small body and head sizes and long limb optima, to be more agile for hunting insects (Losos, 1990; Sanger et al., 2012). Lineages that share a diet type, but have evolved independently across evolutionary history, are expected to also show convergence of ecomorphological traits and body size.
2 Materials and methods
2.1 Taxon sampling and phylogenetic tree
To conduct the analyses using the phylogenetic comparative method, we employed the calibrated tree outlined in Esquerré et al. (2019). This tree is constructed based on six nuclear (B1D, EXPH5, KIF24, MXRA5, PLRL, PNN) and four mitochondrial loci (cytb, 12S, ND2, ND4) as molecular markers. The gene partitioning scheme and substitution model were determined to be GTR+G for optimal accuracy. To establish temporal parameters, a fossil representing the earliest occurrence of the Eulaemus clade in the Early Miocene was incorporated, providing a mean prior for the tree height of this subgenus. Notably, this tree encompasses approximately 66% of the presently identified species within Liolaemidae (Uetz et al., 2023), constituting 1 Ctenoblepharys, 188 Liolaemus, and 35 Phymaturus, totaling 224 species. We utilized data from 187 of these species for which we could find morphometric information.
2.2 Morphological and diet data compilation
Morphological traits used in the analyses included snout–vent length (SVL, 187 species), head length, and head width (HeLe, HeWi, respectively from 178 species), forelimbs, hindlimbs length, length difference between limbs, axilla–groin length (FoLi, HiLi, DiLi, AxGr, respectively from 141 species) (Supplementary Figure 1). Traits were analyzed independently as SVL, head and body measurements, as not all species have information for all the traits analyzed in this study. We mainly used morphological data gathered by one of us (DPD), for which we averaged trait size for males and females independently, and then averaged these two values to obtain a single value for each species. For those species that we did not have measurements, we used published data, i.e., description of the holotype, assuming that this individual is representative of the species. For some species we also used measures reported by Meiri (Meiri, 2018) (Supplementary Table 1). We used diet data reported by Ocampo et al. (2022), but updated with information for Liolaemus polystictus (Olivera-Jara and Aguilar, 2020). For species present in the Esquerré’s et al. (2019) tree, we analyzed the diet of 187 species, which represent 55% of the Liolaemidae family. All data on diet and body measurements can be found in Supplementary Table 2.
2.3 Diet reconstruction
To infer ancestral diet states, we employed Stochastic Character Mapping (SCM) (Bollback, 2006) on the Maximum Clade Credibility (MCC) Phylogenetic Tree. This was achieved using the make.simmap function from the phytools package (Revell, 2012) within the R statistical environment (R Core Team, 2022). SCM, a Bayesian method, utilizes Markov Chain Monte Carlo (MCMC) to produce a posterior probability distribution, grounded in Maximum Likelihood (ML), of ancestral diet states and their transition times across branches on the MCC tree (Huelsenbeck et al., 2003). Before running SCM, we first extracted the 187 species from the Esquerré et al. (2019) tree. With the dietary information for each of these species, we searched for the best model among three possibilities that best fits our data. These models are: 1) an equal-rates model “ER”, where a single parameter governs all transition rates, 2) a symmetric model “SYM”, where forward and reverse transitions share the same parameter, and 3) an all-rates-are-different model “ARD”, where each rate is a unique parameter. These models were assessed using the fitDiscrete function from geiger package, and based on the Akaike Information Criterion (AIC), we selected the best model. The parameters used to run the make.simmap function were: Q=“mcmc”; nsim=500; message=TRUE; model=“SYM”; the rest of the parameters were set to their default values. Model were constructed using 500 simulated trees. We plot the phylogenetic morphospace with the phylomorphospace command from the values obtained with the make.simmap function.
2.4 Morphological evolution
All morphological variables were standardized via log-transformation. To obtain the simmap values and calculate the evolutionary optima from them, a diet reconstruction was performed for each of the three trait trees (explained above), which are: SVL (187 species), head (HeLe, HeWi, 178 species), and body (FoLi, HiLi, DiLi, AxGr, 141 species). To assess if different morphological traits evolved toward different phenotypic optima according to the three diet types, we fitted Ornstein–Uhlenbeck models (OU) of character evolution using the R package OUwie (Beaulieu et al., 2012). We estimated evolutionary optima for all traits for the entire family first, and then separately for the genera Liolaemus and Phymaturus. Initially, we ran the different models with nsim=10 using the make.simmap function and compared their AICc values to identify the set of models with the best fit. Tested models were: a single-rate Brownian motion (BM1), a Brownian motion model with different rate parameters for each state on a tree (BMS), an Ornstein–Uhlenbeck model with a single optimum “θ” for all species (OU1), an Ornstein–Uhlenbeck model with different θ, a single strength of pull “α” and average evolutionary rate “σ2” acting in all selective regimes (OUM), and Ornstein–Uhlenbeck models that assume different state means θ as well as either multiple σ2 (OUMV), multiple α (OUMA), or multiple α and σ2 per selective regime (OUMVA). Once the model with the best fit for each tree was identified, we ran it with all simulations generated by the make.simmap function. For each trait, we also calculated Blomberg’s k phylogenetic signal to assess the independence of the data from their phylogenetic relationships (Blomberg et al., 2003).
For all traits, we performed ancestral reconstruction across lineages (Evans et al., 2009), we employed the phenogram function from the phytools package (Revell, 2012; Revell, 2013) within the statistical environment R (R Core Team, 2022). The position of nodes and branches are computed via ancestral character estimation using likelihood, where each trait evolves according to a Brownian motion process governed by a rate parameter β. Under this model the expected squared difference (variance) between any two species is β multiplied by the time since the species last shared a common ancestor (Schluter et al., 1997). In the case of the remaining body measurements, we corrected for size effects by using the ratio of each trait’s value to snout–vent length.
2.5 Phylogenetic PCA
To compare ecomorphological changes across diets, we conducted a phylogenetic PCA for head and body data separately, considering the difference in tree size. We used logarithmically transformed standardized morphological variables, and corrected for size effects by using the ratio of each trait’s value to snout–vent length, the phylogenetic PCA was performed with the phyl.pca function in the R phytools package (Revell, 2012).
3 Results
3.1 Diet reconstruction
The best evolutionary model for the distribution of characters on the tree according to the three models was “SYM” (ER=341.00, SYM=309.00, ARD=312.13). Diet reconstruction in Liolaemidae shows that the common ancestor is likely to have been omnivorous, (highest probability, p=0.37, compared to an herbivore with p=0.25, or an insectivore with p=0.34; Figure 1A). Similarly, the common ancestor between Phymaturus and Liolaemus is likely to have been omnivorous (highest probability, p=0.38, compared to an herbivore with p=0.27, insectivore p=0.35). From there, Phymaturus transitioned to herbivory and remained as such throughout most of its evolution, with some recent transitions to omnivory (3 species out of 28 analyzed in this study). In Liolaemus, ancestors were primarily insectivores, and omnivory began to reappear convergently across the five main lineages between 9 and 10 Mya. Herbivory in Liolaemus has evolved more recently in the last 4 Mya and has converged ten times within the genus. On mean, 205.17 transitions between diets occurred during the evolution of the family, with insectivory having a higher number of transitions towards omnivory (mean 94.63), followed by omnivory towards insectivory (mean 91.11). Transitions from omnivory to herbivory and vice versa are much lower (11.04 and 6.29 respectively) and the transitions from insectivory to herbivory and vice versa were the lowest of all (1.4 and 0.71 respectively) (Figure 1B). Proportionally, the insectivorous diet has been present for more than half of the evolutionary time (0.51), while the herbivorous diet is a more recent development (0.12). On the other hand, omnivory has been present for a considerable amount of time (0.38).
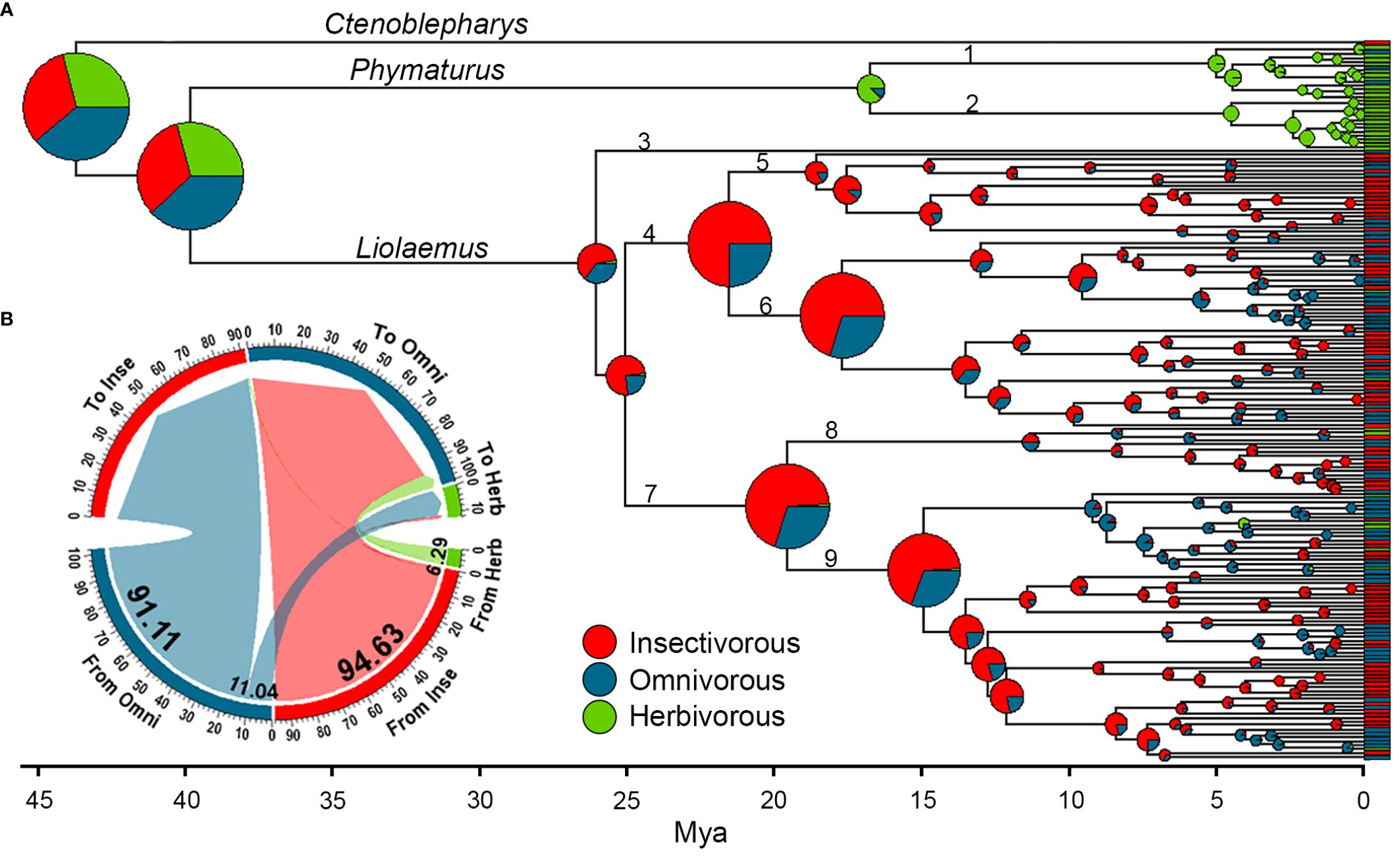
Figure 1 Ancestral reconstruction of dietary diversification throughout the evolutionary history of Liolaemidae (pie charts at nodes represent posterior probabilities of each diet class), averaged across 500 trees. (A) (1) Phymaturus palluma group; (2) Phymaturus patagonicus group; (3) Liolaemus walkeri group; (4) Liolaemus subgenus; (5) Liolaemus nigromaculatus section; (6) Liolaemus chiliensis section; (7) Eulaemus subgenus; (8) Liolaemus lineomaculatus series; (9) Liolaemus montanus series. The probability of the common ancestor being omnivorous is slightly higher than the probabilities of the other two diets. While ancestors of the Liolaemus genus were primarily insectivorous or omnivorous, herbivorous species have independently evolved in different groups within this genus. (B) Mean transitions from one diet type to another, where insectivory had more transitions towards omnivory, followed by a reversal between these two diets. The transitions to and from herbivory are very unlikely, especially the one from herbivory to insectivory, which is represented by only one line with a value of 0.71.
3.2 Morphological evolution
The evolutionary models that best fit the character distribution in the three analyzed trees by genus and by family are presented in Table 1. It can be observed that in the Phymaturus genus, traits are better fitted to a model where all rates are different, whereas in Liolaemus, head traits already exhibit a more symmetric model. This pattern is consistent across all traits analyzed at the family level. Phylogenetic signal and the best-fitted OU models for the traits analyzed by genus and family are presented in Table 2. The values of the OU models are the mean of the 10 runs conducted per trait, in which some instances resulted in an outlier value. This outlier was not considered in the calculation of the mean, and the model that was most frequently repeated was weighted as the best fit. In Phymaturus, the model that best fits all traits is OU1, which means that the different traits evolved towards a single optimum value θ, at a constant rate σ2 and with a similar selective pull α regardless of diet type (Table 3). The phylogenetic signal was low for all traits; however, it was only significant for the SVL and HeWi traits contrary to the rest of the traits (Table 2). For Liolaemus, the model that best fit most traits was also an OU1. However, for HeWi and AxGr, the models that best fit were OUMV and OUMVA, respectively, which exhibited different evolutionary θ, σ2 and α for each diet, indicating that herbivorous species evolved towards wider heads, while insectivorous species evolved towards narrower heads. The highest σ2was for herbivores, and the lowest for omnivores. Similarly, for AxGr the best fit was an OUMVA model with different θ, σ2, and α for each diet (Table 3). In this genus, a low phylogenetic signal was found for all traits (Table 2).
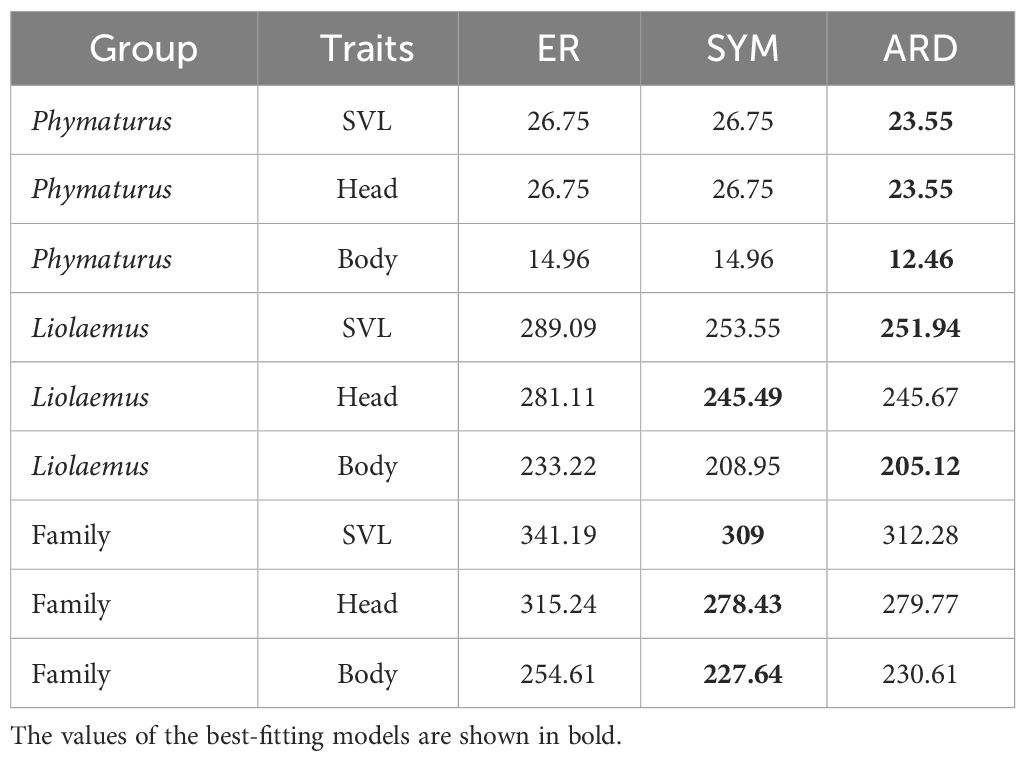
Table 1 Results of the model comparison, indicating the best fit based on the obtained AIC values, were conducted for the SVL, head (HeLe, HeWi), and body (FoLi, HiLi, DiLi, AxGr) trees, both for the Phymaturus and Liolaemus genera, as well as an analysis of the entire Liolaemidae family.
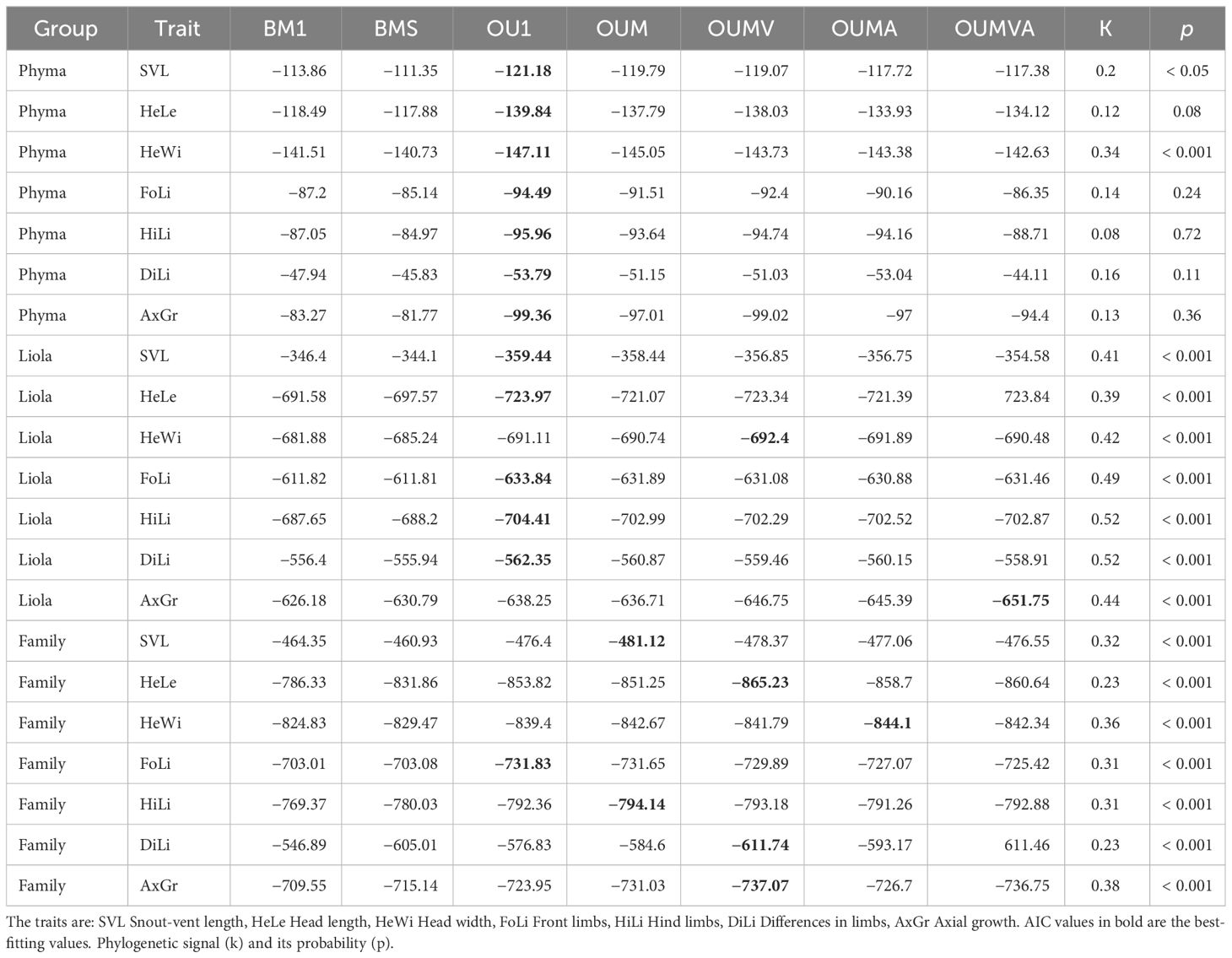
Table 2 Mean of the AIC values obtained for each OU models for the traits analyzed by genus (PHYMA, Phymaturus; LIOLA, Liolaemus) and by family.
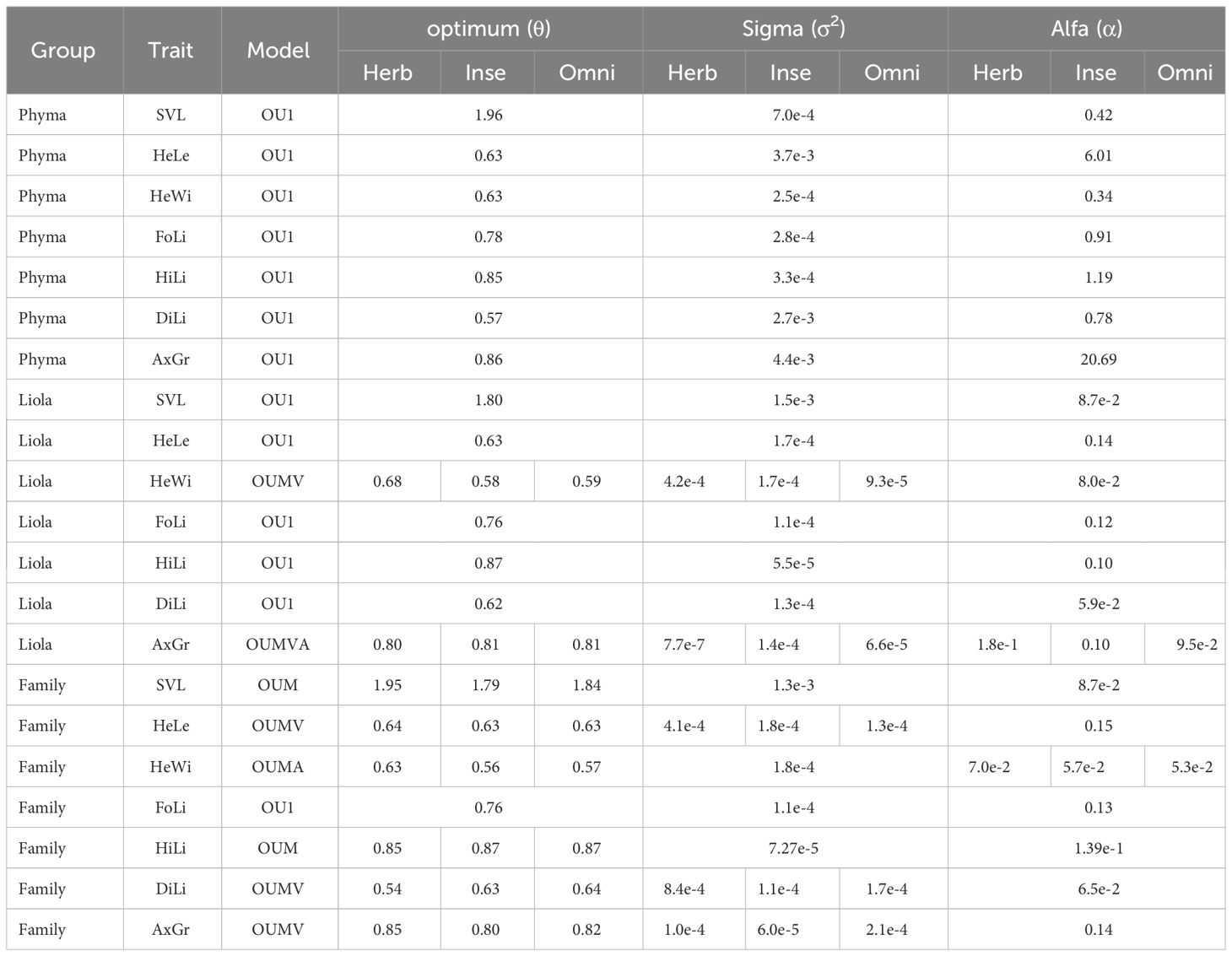
Table 3 Optimal values, sigma, and alpha obtained in the OUwie analysis by genus (PHYMA, Phymaturus; LIOLA, Liolaemus) and by family. Phymaturus exhibits a single optimum for all its traits, while Liolaemus begins to show some traits that have more than one evolutionary optimum. However, at the family level, almost all traits present optima for each type of diet.
In the analysis of the Liolaemidae family, most of the traits exhibit a θ for each diet type, except FoLi that showed a single θ for all diet types (Table 3). The SVL has a well-defined θ for each diet type, the herbivorous species reached an evolutionary optimum at 87 mm of SVL, while the omnivores reach their evolutionary optimum at 70 mm, and the insectivores at 57 mm (Figure 2). A trait that showed different θ´s and σ2s for each diet was HeLe, where herbivores have longer head and insectivores have shorter head, while σ2 was highest for herbivores, and the lowest for omnivores (Figure 3A). Only HeWi exhibited significantly different θs and αs, where herbivores have wider heads and insectivores have narrower ones; the highest α was for herbivores, and the lowest for omnivores (Figure 3B), DiLi where omnivores and insectivores have the greatest θ´s difference between their fore and hind limbs, while herbivores have the smallest difference. The highest σ2 was for herbivores, and the lowest for insectivores (Figure 3C), AxGr where herbivores have the greatest axial growth, and insectivores have the lowest. The highest σ2 was for omnivores, and the lowest for insectivores (Figure 3D). HiLi only exhibits differences in θ´s where insectivores have longer Hind limbs and herbivores have shorter Hind limbs (Figure 3E), In most traits, the phylogenetic signal was significantly low (p < 0.001), indicating a general tendency for less phylogenetic signal than expected under Brownian motion. Only in the genus Phymaturus, the traits HeLe, FoLi, HiLi, DiLi, AxGr were not significant (Table 2).
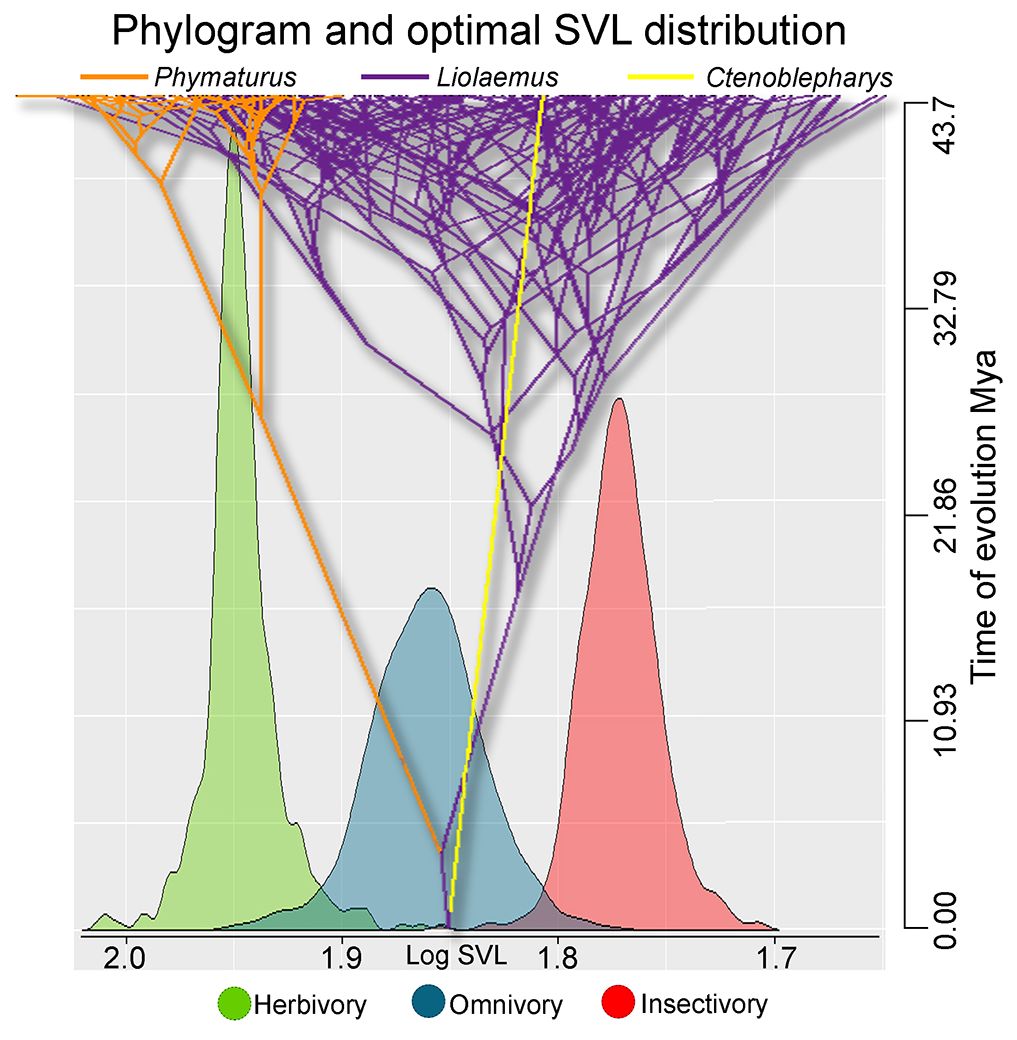
Figure 2 Snout–vent length (SVL) evolution in Liolaemidae, phenogram of the ancestral reconstruction of SVL where the values on the x-axis are in logarithm, and the y-axis shows the evolution time of the trait. Histograms are the values of the optimal sizes θ for each diet estimated with Ornstein–Uhlenbeck models. The ancestral reconstruction of the (SVL) of the common ancestor of the family, suggests that it may have had a size very close to the optimal value for omnivorous species. There is a significant variation in size ranges between the genera Phymaturus and Liolaemus, indicating that the latter has acquired a wide variety of sizes beyond the optimal ranges.
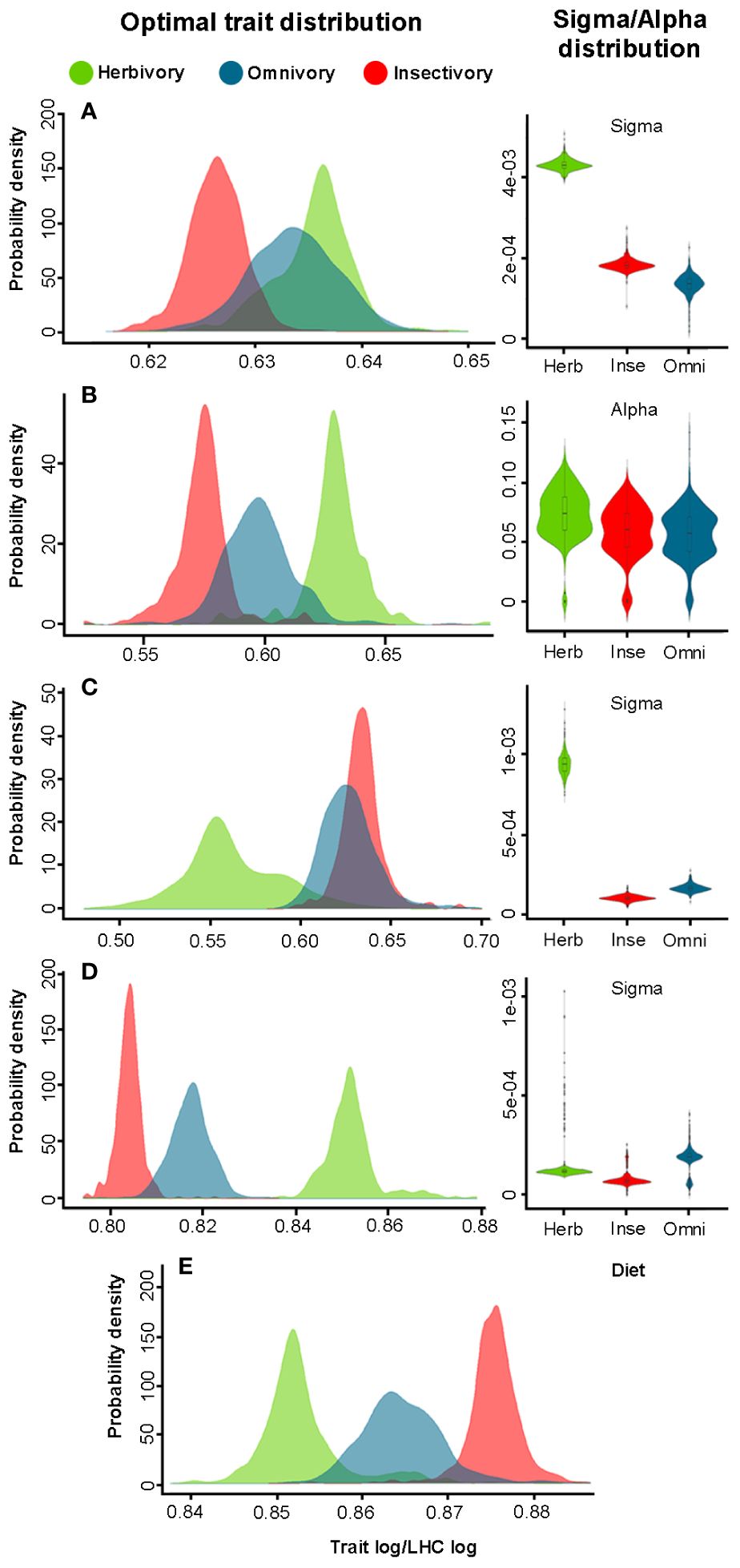
Figure 3 Differences in θ, σ2, and α among three types of diets in Liolaemidae, where (A) is head length, (B) Head width, (C) differences in limbs, (D) axial growth length, (E) hindlimbs length. Herbivorous species exhibit larger heads and bodies, while their limbs are smaller. Her σ2 rate was higher in head and limb traits, as well as the selective force α on head width.
The Liolaemidae family currently encompasses a wide range of body sizes across lineages, from the large (112.1 mm) to the small (44.7 mm) species, representing a 2.7-fold increase in size. The SVL reconstruction shows that the ancestor of the family may have had around 71 mm of SVL (Figure 2), very close to the optimal size for omnivores. This result supports the likelihood that the common ancestor could have been omnivorous. Additionally, the rest of the traits tend to show the same outcome, except for hind limb length (Supplementary Figure 2). Phymaturus evolved to the largest sizes, Ctenoblepharys to a lower medium size, and Liolaemus evolved a wide range of sizes showing the greatest variation in SVL of the three genera.
3.3 Phylogenetic PCA
Phylogenetic PCA shows that herbivory has a particular body and head shape that differentiates it from omnivory and insectivory (Figure 4). In the head analysis, the principal component 1 explains 87% of the variation, and both head width and length are good traits to separate groups. In the results of the body the principal component 1 explains 58% of the variation, and difference between limbs is a good trait to separate groups (Table 4).
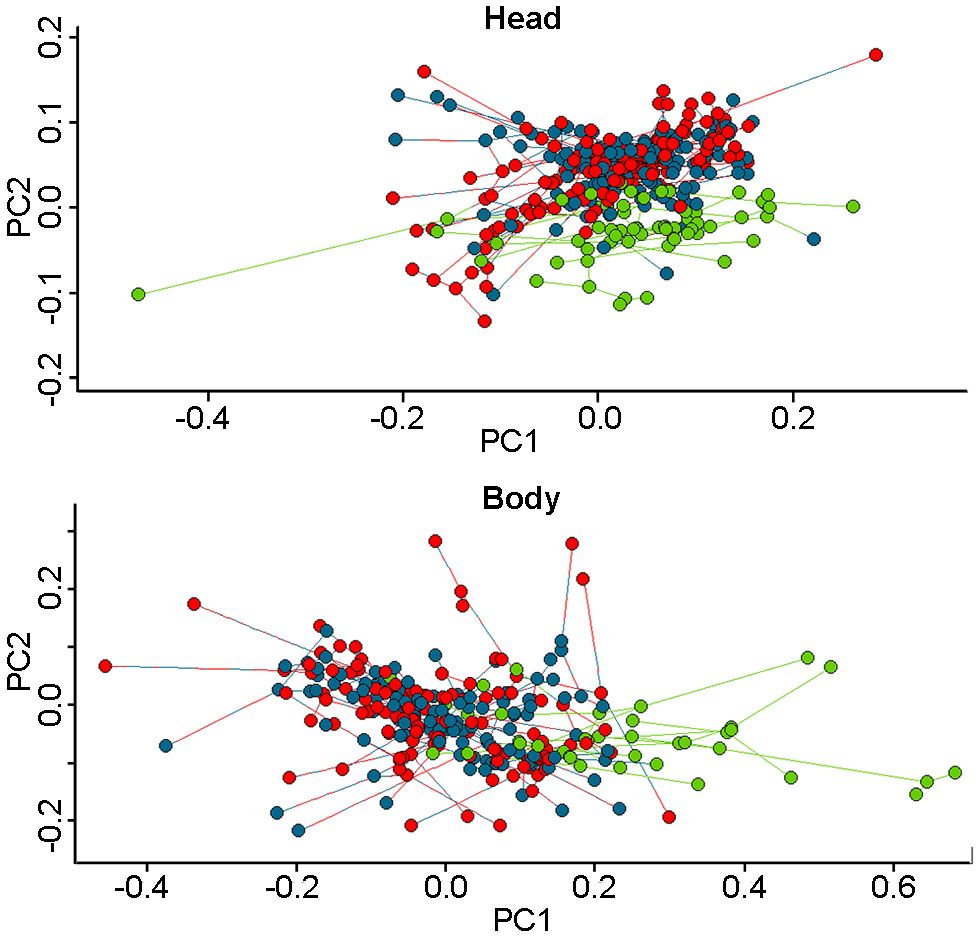
Figure 4 Phylogenetic morphospace of the traits measured in the head and body of the different species of the Liolaemidae family. Green points represent herbivorous species, blue points omnivorous, and red points insectivorous. In both graphs, herbivorous species tend to diverge from the other diets, while insectivores and omnivores do not show significant morphological differences.
4 Discussion
Our findings elucidate that the evolution of distinct dietary regimes steered the Liolaemidae family towards optimal head and body morphologies, underscoring the presence of both adaptive and non-adaptive evolutionary processes in size and body form within its two most abundant genera. These evolutionary optima manifest with greater clarity when considering the entire family. Herbivorous species emerge as the lizard subgroup exhibiting the most pronounced morphological variations attributable to dietary adaptation. Head width emerges as a pivotal trait, notably distinguished by its differential response to interacting selective forces.
Our results support the hypothesis that diet evolution has driven the evolution of three optimal body sizes in the family. Pincheira-Donoso et al. (2015) previously examined body size in the genus Liolaemus in search of an adaptive radiation pattern. They found that this trait was best explained by an OU stabilizing selection model with three distinct evolutionary optima (e.g., OUM). These three optimal body sizes closely align with our results, differing only in the central value. In our analysis, it corresponds to the size of omnivorous diets (70 mm in this study compared to 80 mm in Pincheira-Donoso et al., 2015). It’s worth noting that their study focused solely on the Liolaemus genus (109 species), yet our analysis encompasses all three genera and a larger portion of the family (187 species). Therefore, the difference in SVL optima for omnivores could potentially be attributed to sample size bias. In this context Cooper and Vitt (2002) examined herbivory and body size across 450 species from 23 lizard families, although their primary goal was not to identify evolutionary optima, their results indicated that the majority of herbivorous species closely approached the optimum size value we report here (Cooper Jr. and Vitt, 2002). Similarly, Van Damme (1999) analyzed 97 populations representing 52 species within the Lacertidae family and found body size averages very much in line with our findings for herbivorous and insectivorous diets (Van Damme, 1999). These results suggest that the Liolaemidae family can serve as a representative model for lizards in general.
Reconstruction of the evolution of diet in Liolaemidae showed that the common ancestor of the family is likely to have been omnivorous, challenging what was found previously by other authors (Espinoza et al., 2004; Ocampo et al., 2022). This result might be far from conclusive, because probabilities of the three diet types at the ancestral node are very similar in value. In addition, the combination of diet and genetic data of almost half of the Liolaemidae family has yet to be completed for definitive analysis and conclusion. However, our reconstruction of the SVL size of the common ancestor (55% representativeness) support our result that the ancestor could have been omnivorous, increasing this probability, and this makes a lot of sense, since there is more likely to be a transition from omnivore to herbivore or insectivore, allowing the divergence of the family in three genera with different diets (Ocampo et al., 2022).
The analyses of evolutionary optima in SVL related to diet in Liolaemus and Phymaturus (analyzed separately) reveal distinct patterns of radiation within each genus. Phymaturus showed evidence of widespread evolution toward a single optimum in morphology and body size in relation to diet, exhibiting lack of clade variation in resource usage. This finding aligns with previous studies that indicate minimal ecological differentiation in reproduction coupled with limited dispersal ability (Scolaro et al., 2013; Pincheira-Donoso et al., 2015; Scolaro et al., 2016; Reaney et al., 2018). Altogether, these patterns support the evidence of non-adaptive radiation observed in their range distribution and habitat use (Scolaro and Pincheira-Donoso, 2010). This genus is nearly invariably viviparous, herbivorous, and saxicolous, with species often isolated due to their dependence on these rocky outcrops as their habitat (Ibargüengoytía et al., 2008; Díaz, 2009). Conversely, Liolaemus shows multiple optimal sizes associated to head width in relation to each diet type which accounts for the great variation in resource use within the genus. The head represents a remarkably complex part of the body due to its multifunctionality, encompassing feeding, breathing, vision, chemoreception, defense, combat, sexual attraction, brain protection, and communication (Herrel et al., 2001; Lappin and Husak, 2005; Kohlsdorf et al., 2008). For all these functions, the head is subject to diverse and intricate selective forces that may sometimes be in conflict (Kohlsdorf et al., 2008). The optimal head shape observed in herbivores in this study aligns with the morphological requirements for acquiring and consuming plant material, which is characterized by large heads (Herrel, 2007). The pterygoid, the largest jaw muscle in lizards (Gröning et al., 2013), provides substantial bite force and is highly developed in herbivorous species, enabling efficient reaping of tough, fibrous items such as leaves (Herrel, 2007). In contrast, insectivorous species exhibit smaller heads, offering less bite force but potentially enhancing agility for capturing mobile prey (Herrel, 2007). On the other hand, the distinct optima found in axial development for each dietary type in Liolaemus are accompanied by a clear separation of herbivorous species from the other diets. This trait developed at a slower rate but with higher selective pressure. Selective pressures interacting with body size can be of various types and may be in opposition, but they can also be related to the type of food consumed (Tennis et al., 1979; Winne et al., 2010). Liolaemus exhibits greater ecological differentiation in its reproduction, diet, habitat use, dispersal among others (Pincheira-Donoso, 2011; Pincheira-Donoso et al., 2015; Edwards et al., 2022). Therefore, it is undoubtedly a completely different type of radiation than Phymaturus. The great ecological differences related to resource use as basis for divergent selection, couple with the frequent convergence in morphology and body size associated with diet evolution, further supports an adaptive radiation-based diversification of the genus. This opens up a huge possibility for further evolutionary studies using this family as an example group given the contrasting evolutionary patterns found across clades.
In the family, most traits exhibited a higher rate of change (σ2) in herbivores, possibly due to increased selective pressure, as indicated by our results on head width. This finding is of particular interest as it supports the hypothesis that head morphology evolves more rapidly than overall body shape, a pattern observed in the evolutionary history of various species, including tyrannosaurids, geckos, and finches (Grant and Grant, 2002; Stokstad, 2004; Eloy de Amorim et al., 2017). Another example of this pattern can be found in human evolution, where changes in brain size and reductions in bite force due to dietary shifts have significantly influenced body shape and size (Aiello and Wheeler, 1995; Lieberman, 2011). Changes in evolutionary rate (i.e., σ2) with a change in diet can trigger a series of morphological and physiological adaptations, including an extended intestinal length (Pincheira-Donoso, 2021); including the presence of colonic valves in the intestines, which slow down food passage to facilitate digestion by the necessary intestinal flora for cellulose degradation (Iverson, 1982), and symbiotic associations with other organisms (bacteria and protozoa) aiding in the digestion of plant material (Cooper Jr. and Vitt, 2002; Espinoza et al., 2004).
The relatively high variation observed between front and hind limbs is indicative of the need to change body morphology to adopt a novel foraging strategies and habitat utilization with a change in diet (Miles et al., 2007). Our findings indicate that herbivorous species tend to adapt towards shorter limbs with minimal variation between them. An herbivorous diet often involves less active food searching (Cooper Jr., 1995). Conversely, omnivorous and insectivorous species exhibit longer hind limbs, which enable them to run faster and capture mobile prey, sometimes even resorting to bipedal locomotion (Losos, 1990; Irischick and Jayne, 1999; Aerts et al., 2003; Miles et al., 2007). Notably, omnivores display longer forelimbs, which could represent an intermediate stage between insectivores and herbivores. According to our results, they seem to be in the process of reducing the variation between forelimbs and hind limbs to achieve the body proportions of herbivores. The transition from insectivory to herbivory through omnivory was also reported by Ocampo et al. (2022). Losos et al. (2000) have proposed that limb length can be influenced by various factors, including differences in microhabitat utilization, given its considerable phenotypic plasticity (Losos et al., 2000). It is important to note, however, that intrapopulation variation of a trait typically does not surpass the species baseline morphology. Furthermore, this trait in limb length may be subject to strong exaptation, representing a product of an original and suitable function (Revell et al., 2007) such as dietary adaptation.
The phylogenetic PCA highlights significant differences in head and body morphology from herbivorous species compared to the other two diets, underscoring the implications of physical and physiological adaptations associated with this dietary type (Iverson, 1982; Espinoza et al., 2004; Ocampo et al., 2022). The shift to herbivory represented a pivotal innovation within the family, enabling them to exploit new ecological niches in their environment (Miller et al., 2022). Notably, aside from SVL, traits of omnivorous species closely resemble those of insectivores. This observation may be linked to the rapid and drastic morphological changes in the head that accompany the transition to herbivory, while changes in size occur more gradually.
5 Conclusion
Our findings underscore the significant role of diet in shaping the evolution of body form within the Liolaemidae lizard family, giving rise to distinct optimal body shapes corresponding to each dietary preference. While factors such as sexual selection, predation, competition, and others also exert considerable influence on this process, diet emerges as a consistent and prevalent driver in this group. The influence of diet evolution and the evolution of Liolaemidae can be observed when we compare diet evolution at the clade level (i.e., genus level). When diet change very little though evolution, such as in Phymaturus, the is low morphological variation and the high diversification does not reflect adaptive radiation. Phymaturus has been previously described as a genus that evolved through non-adaptive radiation (Reaney et al., 2018). On the contrary, when diet evolution shows great diversity (e.g., the evolution of three diet types), it is repeatably convergent and has generated great morphological and size variation across species, high diversification is linked to adaptive radiation vis a vis the use of food resources.
The rapid evolution of head relative to body length in herbivores presents a compelling avenue for future research. Furthermore, the remarkable diversity within the Liolaemidae family, coupled with its broad spectrum of habitat utilization, positions it as a valuable representation of the entire lizard group.
Data availability statement
The original contributions presented in the study are included in the article/Supplementary Material. Further inquiries can be directed to the corresponding author.
Author contributions
MO: Conceptualization, Data curation, Formal analysis, Investigation, Methodology, Visualization, Writing – original draft. DP: Conceptualization, Supervision, Writing – review & editing. RR: Conceptualization, Methodology, Supervision, Writing – review & editing, Formal Analysis.
Funding
The author(s) declare that no financial support was received for the research, authorship, and/or publication of this article.
Acknowledgments
We would like to thank Lizette Siles for comments that greatly improved the manuscript.
Conflict of interest
The authors declare that the research was conducted in the absence of any commercial or financial relationships that could be construed as a potential conflict of interest.
Publisher’s note
All claims expressed in this article are solely those of the authors and do not necessarily represent those of their affiliated organizations, or those of the publisher, the editors and the reviewers. Any product that may be evaluated in this article, or claim that may be made by its manufacturer, is not guaranteed or endorsed by the publisher.
Supplementary material
The Supplementary Material for this article can be found online at: https://www.frontiersin.org/articles/10.3389/fevo.2024.1361799/full#supplementary-material
References
Abdala C. S., Quinteros A. S. (2014). Los últimos 30 años de estudios de la familia de lagartijas más diversa de Argentina. Actualización taxonómica y sistemática de Liolaemidae. Cuadernos Herpetología 28, 55–82.
Aerts P., Van Damme R., D’Aout K., Van Hooydonck B. (2003). Bipedalism in lizards: whole-body modelling reveals a possible spandrel. Philos. Trans. R. Soc. London B 358, 1525–1533. doi: 10.1098/rstb.2003.1342
Aiello L. C., Wheeler P. (1995). The expensive-tissue hypothesis, The brain and the digestive system in human and primate evolution. Curr. Anthropology 36, 199–221. doi: 10.1086/204350
Aparicio J., Ocampo M. (2010). Liolaemus grupo montanus Etheridge 1995 (Iguania - liolaemidae). Cuadernos Herpetología 24, 133–135.
Astudillo-Clavijo V., Arbour J. H., López-Fernández H. (2015). Selection towards different adaptive optima drove the early diversification of locomotor phenotypes in the radiation of Neotropical geophagine cichlids. BMC Evolutionary Biol. 15, 77. doi: 10.1186/s12862-015-0348-7
Beaulieu J. M., Jhwueng D.-C., Boettiger C., O’Meara B. C. (2012). Modeling stabilizing selection: expanding the Ornstein–Uhlenbeck model of adaptive evolution. Evolution 66, 2369–2383. doi: 10.1111/evo.2012.66.issue-8
Blomberg S. P., Garland T. Jr., Ives A. R. (2003). Testing for phylogenetic signal in comparative data: behavioral traits are more labile. Evolution 57, 717–745. doi: 10.1111/j.0014-3820.2003.tb00285.x
Bollback J. P. (2006). SIMMAP: Stochastic character mapping of discrete traits on phylogenies. BMC Bioinf. 7, 88. doi: 10.1186/1471-2105-7-88
Burin G., Kissling W. D., Guimaraes P. R., Sekercioglu C. H., Quental T. B. (2016). Omnivory in birds is a macroevolutionary sink. Nat. Commun. 7, 11250. doi: 10.1038/ncomms11250
Cerdeña J., Farfán J., Quiroz A. J. (2021). A high mountain lizard from Peru: The world’s highest-altitude reptile. Herpetozoa 34, 61–65. doi: 10.3897/herpetozoa.34.61393
Clauss M., Steuer P., Müller D. W. H., Codron D., Hummel J. (2013). Herbivory and body size: allometries of diet quality and gastrointestinal physiology, and implications for herbivore ecology and dinosaur gigantism. PloS One 8, e68714. doi: 10.1371/journal.pone.0068714
Cooper (1995). Foraging mode, prey chemical discrimination, and phylogeny in lizards. Anim. Behav. 50, 973–985. doi: 10.1016/0003-3472(95)80098-0
Cooper W. E. Jr., Vitt L. J. (2002). Distribution, extent, and evolution of plant consumption by lizards. J. Zoology 257, 487–517. doi: 10.1017/S0952836902001085
Czekanski-Moir J. E., Rundell R. J. (2019). The ecology of nonecological speciation and nonadaptive radiations. Trends Ecol. Evol. 34, 400–415. doi: 10.1016/j.tree.2019.01.012
Díaz G. J. M. (2009). Historical biogeography of Phymaturus (Iguania: Liolaemidae) from Andean and patagonian South America. Zoologica Scripta 38, 1–7. doi: 10.1111/j.1463-6409.2008.00357.x
Dollion A. Y., Measey G. J., Cornette R., Liza C., Tolley K. A., da Silva J. M., et al. (2017). Does diet drive the evolution of head shape and bite force in chameleons of the genus Bradypodion? Funct. Ecol. 31, 671–684. doi: 10.1111/1365-2435.12750
Edwards D. L., Avila L. J., Martinez L., Sites J. W. Jr., Morando M. (2022). Environmental correlates of phenotypic evolution in ecologically diverse Liolaemus lizards. Ecol. Evol. 12, e9009. doi: 10.1002/ece3.9009
Eloy de Amorim M., Schoener T. W., Santoro G. R. C. C., Ramalho L. A. C., Piovia-Scott J., Albuquerque B. R. (2017). Lizards on newly created islands independently and rapidly adapt in morphology and diet. Proceeding Natl. Acad. Sci. 114 (33), 8812–8816. doi: 10.1073/pnas.1709080114
Espinoza R. E., Wiens J. J., Tracy C. R. (2004). Recurrent evolution of herbivory in small, cold-climate lizards: Breaking the ecophysiological rules of reptilian herbivory. Proceeding Natl. Acad. Sci. 101, 16819–16824. doi: 10.1073/pnas.0401226101
Esquerré D., Brennan I. G., Catullo R. A., Torres-Pérez F., Keogh J. S. (2019). How mountains shape biodiversity: The role of the Andes in biogeography, diversification, and reproductive biology in South America’s most species-rich lizard radiation (Squamata: Liolaemidae). Evolution 73, 214–230. doi: 10.1111/evo.13657
Evans M. E. K., Smith S. A., Flynn R. S., Donoghue M. J. (2009). Climate, niche evolution, and diversification of the “Bird-cage” Evening primroses (Oenothera, sections anogra and kleinia). Am. Nat. 173, 225–240. doi: 10.1086/595757
Gearty W., McClain C. R., Payne J. L. (2018). Energetic tradeoffs control the size distribution of aquatic mammals. Proceeding Natl. Acad. Sci. 115, 4194–4199. doi: 10.1073/pnas.1712629115
Grant P. R., Grant B. R. (2002). Unpredictable evolution in a 30-year study of darwin’s finches. Science 296, 707–711. doi: 10.1126/science.1070315
Grant B. R., Grant P. R. (2003). What Darwin’s finches can teach us about the evolutionary origin and regulation of biodiversity. BioScience 53, 965–975. doi: 10.1641/0006-3568(2003)053[0965:WDFCTU]2.0.CO;2
Gröning F., Jones M. E. H., Curtis N., Herrel A., O’Higgins P., Evans S. E., et al. (2013). The importance of accurate muscle modelling for biomechanical analyses: a case study with a lizard skull. J. R. Soc. Interface 10, 20130216. doi: 10.1098/rsif.2013.0216
Herrel A. (2007). “Herbivory and foraging mode in lizards,” in Lizard ecology, the evolutionary consequences of foraging mode. Eds. Reilly S. M., McBrayer L. D., Miles D. B. (Cambridge University Press, New York), 209–236.
Herrel A., M. V., De Vree F. (1999). Modulatory complexity of the feeding repertoire in scincid lizards. J. Comp. Physiol. A 184, 501–518. doi: 10.1007/s003590050350
Herrel A., Meyers J. J., Vanhooydonck B. (2001). Correlations between habitat use and body shape in a phrynosomatid lizard (Urosaurus ornatus): a population-level analysis. Biol. J. Linn. Soc. 74, 305–314. doi: 10.1006/bijl.2001.0579
Herrel A., Vanhooydonck B., Van Damme R. (2004). Omnivory in lacertid lizards: adaptive evolution or constraint? J. Evolutionary Biol. 17, 974–984. doi: 10.1111/j.1420-9101.2004.00758.x
Huelsenbeck J. P., Nielsen R., Bollback J. P. (2003). Stochastic mapping of morphological characters. Systematic Biol. 52, 131–158. doi: 10.1080/10635150390192780
Ibargüengoytía N. R., Acosta J. C., Boretto J. M., Villavicencio H. J., Marinero J. A., Krenz J. D. (2008). Field thermal biology in Phymaturus lizards: Comparisons from the Andes to the Patagonian steppe in Argentina. J. Arid Environments 72, 1620–1630. doi: 10.1016/j.jaridenv.2008.03.018
Irischick D. J., Jayne B. C. (1999). Comparative three-dimensional kinematics of the hindlimb for high-speed bipedal and quadrupedal locomotion of lizards. J. Exp. Biol. 202, 1047–1065. doi: 10.1242/jeb.202.9.1047
Iverson J. B. (1982). “Adaptations to herbivory in iguanine lizards,” in Iguanas of the world. Their behavior, ecology, and conservation. Eds. Burghardt G. M., Rand A. S. (Noyes Publications, New Jersey), 60–76.
Jones A. W., Palkovacs E. P., Post D. M. (2013). Recent parallel divergence in body shape and diet source of alewife life history forms. Evolutionary Ecol. 27, 1175–1187. doi: 10.1007/s10682-013-9650-2
Kohlsdorf T., Grizante M. B., Navas C. A., Herrel A. (2008). Head shape evolution in Tropidurinae lizards: does locomotion constrain diet? J. Evolutionary Biol. 21, 781–790. doi: 10.1111/j.1420-9101.2008.01516.x
Lapiedra O., Sayol F., Garcia-Porta J., Sol D. (2021). Niche shifts after island colonization spurred adaptive diversification and speciation in a cosmopolitan bird clade. Proceding R. Soc. B 288, 20211022. doi: 10.1098/rspb.2021.1022
Lapiedra O., Sol D., Carranza S., Beaulieu J. M. (2013). Behavioural changes and the adaptive diversification of pigeons and doves. Proceding R. Soc. B 280, 1–9. doi: 10.1098/rspb.2012.2893
Lappin A. K., Husak J. F. (2005). Weapon performance, not size, determines mating success and potential reproductive output in the Collared Lizard (Crotaphytus collaris). Am. Nat. 166, 426–436. doi: 10.1086/432564
Lieberman D. E. (2011). The evolution of the human head (Massachusetts: Harvard University). doi: 10.4159/9780674059443
Losos J. B. (1990). The evolution of form and function: morphology and locomotor performance in west Indian Anolis lizards. Evolution 44, 1189–1203. doi: 10.2307/2409282
Losos J. B., Creer D. A., Glossip D., Goellner R., Hampton A., Roberts G., et al. (2000). Evolutionary implications of phenotypic plasticity in the hindlimb of the lizard Anolis sagrei. Evolution 54, 301–305. doi: 10.1554/0014-3820(2000)054[0301:EIOPPI]2.0.CO;2
Mahler D. L., Ingram T., Revell L. J., Losos J. B. (2013). Exceptional convergence on the macroevolutionary landscape in island lizard radiations. SCIENCE 341, 292–295. doi: 10.1126/science.1232392
Matsubayashi K. W., Yamaguchi R. (2020). The speciation view: Disentangling multiple causes of adaptive and non-adaptive radiation in terms of speciation. Population Ecol. 64, 95–107. doi: 10.1002/1438-390X.12103
Mazel F., Wüest R. O., Gueguen M., Renaud J., Ficetola G. F., Lavergne S., et al. (2017). The geography of ecological niche evolution in mammals. Curr. Biol. 27, 1–6. doi: 10.1016/j.cub.2017.03.046
Meiri S. (2018). Traits of lizards of the world: Variation around a successful evolutionary design. Global Ecol. Biogeography 27, 1168–1172. doi: 10.1111/geb.12773
Miles D. B., Losos J. B., Irischick D. J. (2007). “Morphology, performance, and foraging mode,” in Lizard ecology. Eds. Reilly S. M., Mc Brayer L. B., Miles. D. B. (Cambridge University Press, New York), 49–93.
Miller A. H., Stroud J. T., Losos J. B. (2022). The ecology and evolution of key innovations. Trends Ecol. Evol. 38 (2), 122–131. doi: 10.1016/j.tree.2022.09.005
Muschick M., Indermaur A., Salzburger W. (2012). Convergent evolution within an adaptive radiation of Cichlid Fishes. Curr. Biol. 22, 2362–2368. doi: 10.1016/j.cub.2012.10.048
Ocampo M., Pincheira-Donoso D., Sayol F., Rios R. S. (2022). Evolutionary transitions in diet infuence the exceptional diversifcation of a lizard adaptive radiation. BMC Ecol. Evol. 22, 74. doi: 10.1186/s12862-022-02028-3
O’Grady S. P., Morando M., Avila L., Dearing M. D. (2005). Correlating diet and digestive tract specialization: Examples from the lizard family Liolaemidae. Zoology 108, 201–210. doi: 10.1016/j.zool.2005.06.002
Olivera-Jara D. A., Aguilar C. A. (2020). Dieta de la lagartija neotropical Liolaemus polystictus (Squamata: Liolaemidae) de los andes centrales, Huancavelica, Perú. Rev. Peruana biología 27, 339–348. doi: 10.15381/rpb.v27i3.18680
O’Meara B. C., Beaulieu J. M. (2014). “Modelling stabilizing selection: the attraction of ornstein–uhlenbeck models,” in Modern phylogenetic comparative methods and their application in evolutionary biology: concepts and practice. Ed. Garamszegi L. Z. (Springer, New York), 552.
Pincheira-Donoso D. (2011). Predictable variation of range-sizes across an extreme environmental gradient in a lizard adaptive radiation: evolutionary and ecological inferences. PloS One 6, 1–8. doi: 10.1371/journal.pone.0028942
Pincheira-Donoso D. (2021). Correlated evolution between herbivory and gastrointestinal tract in a prolific lizard adaptive radiation. Anim. Biol. 71, 233–241. doi: 10.1163/15707563-bja10051
Pincheira-Donoso D., Harvey L. P., Ruta M. (2015). What defines an adaptive radiation? Macroevolutionary diversification dynamics of an exceptionally species-rich continental lizard radiation. BMC Evolutionary Biol. 15, 1–13. doi: 10.1186/s12862-015-0435-9
Pincheira-Donoso D., Hodgson D. J., Jan S., Tregenza T. (2009). A phylogenetic analysis of sex-specific evolution of ecological morphology in Liolaemus lizards. Ecol. Res. 24 (6), 1223–1231. doi: 10.1007/s11284-009-0607-4
Pincheira-Donoso D., Hodgson D. J., Tregenza T. (2008a). The evolution of body size under environmental gradients in ectotherms: why should Bergmann’s rule apply to lizards? BMC Evolutionary Biol. 8, 1–13. doi: 10.1186/1471-2148-8-68
Pincheira-Donoso D., Jara M., Reaney A., García-Roa R., Saldarriaga-Córdoba M., Hodgson D. J. (2017). Hypoxia and hypothermia as rival agents of selection driving the evolution of viviparity in lizards. Global Ecol. Biogeography 26, 1238–1246. doi: 10.1111/geb.12626
Pincheira-Donoso D., Scolaro J. A., Sura P. (2008b). A monographic catalogue on the systematics and phylogeny of the South American iguanian lizard family Liolaemidae (Squamata, Iguania). Zootaxa 1800, 1–85. doi: 10.11646/zootaxa.1800.1
Pincheira-Donoso D., Tregenza T. (2011). Fecundity selection and the evolution of reproductive output and sex-specific body size in the Liolaemus lizard adaptive radiation. Evolutionary Biol. 38, 197–207. doi: 10.1007/s11692-011-9118-7
Pincheira-Donoso D., Tregenza T., Butlin R. K., Hodgson D. J. (2018). Sexes and species as rival units of niche saturation during community assembly. Global Ecol. Biogeography 27, 593–603. doi: 10.1111/geb.12722
Pincheira-Donoso D., Tregenza T., Witt M. J., Hodgson D. J. (2013). The evolution of viviparity opens opportunities for lizard radiation but drives it into a climatic cul-de-sac. Global Ecol. Biogeography 22 (7), 857–867. doi: 10.1111/geb.12052
Price S. A., Hopkins S. S. B., Smith K. K., Roth V. L. (2012). Tempo of trophic evolution and its impact on mammalian diversification. Proceeding Natl. Acad. Sci. 109, 7008–7012. doi: 10.1073/pnas.1117133109
R Core Team (2022). R: A language and environment for statistical computing. 4.2.1 (Vienna, Austria: R Foundation for Statistical Computing).
Reaney A. M., Saldarriaga-Córdoba M., Pincheira-Donoso D. (2018). Macroevolutionary diversification with limited niche disparity in a species-rich lineage of cold-climate lizards. BMC Evolutionary Biol. 18, 1–12. doi: 10.1186/s12862-018-1133-1
Revell L. J. (2012). Application phytools: an R package for phylogenetic comparative biology (and other things). Methods Ecol. Evol. 3, 217–223. doi: 10.1111/j.2041-210X.2011.00169.x
Revell L. J. (2013). Two new graphical methods for mapping trait evolution on phylogenies. Methods Ecol. Evol. 4, 754–759. doi: 10.1111/2041-210X.12066
Revell L. J., Johnson M. A., Schulte J. A. II, Kolbe J. J., Losos J. B. (2007). A phylogenetic test for adaptive convergence in rock-dwelling lizards. Evolution 61, 2898–2912. doi: 10.1111/j.1558-5646.2007.00225.x
Roll U., Feldman A., Novosolov M., Allison A., Bauer A. M., Bernard R., et al. (2017). The global distribution of tetrapods reveals a need for targeted reptile conservation. Nat. Ecol. Evol. 1, 1677–1682. doi: 10.1038/s41559-017-0332-2
Rundell R. J., Price T. D. (2009). Adaptive radiation, nonadaptive radiation, ecological speciation and nonecological speciation. Trends Ecol. Evol. 24, 394–399. doi: 10.1016/j.tree.2009.02.007
Sanger T. J., Mahler D. L., Abzhanov A., Losos J. B. (2012). Roles for modularity and constraint in the evolution of cranial diversity among Anolis lizards. Evolution 66, 1525–1542. doi: 10.1111/j.1558-5646.2011.01519.x
Schluter D. (2000). The ecology of adaptive radiation (New York: Oxford University Press). doi: 10.1093/oso/9780198505235.001.0001
Schluter D., Price T., Mooers A.Ø., Ludwig D. (1997). Likelihood of ancestor states in adaptive radiation. Evolution 51, 1699–1711. doi: 10.2307/2410994
Scolaro J. A., Corbalán V., Tappari O. F. (2016). Lizards at the end of the world: A new melanic species of Phymaturus of the patagonicus clade from rocky outcrops in the northwestern steppe of Chubut province, Patagonia Argentina (Reptilia: Iguania: Liolaemidae). Boletín del Museo Nacional Hist. Natural Chile 65, 137–152.
Scolaro J. A., Jara M., Pincheira-Donoso D. (2013). The sexual signals of speciation? A new sexually dimorphic Phymaturus species of the patagonicus clade from Patagonia Argentina. Zootaxa 3722, 317–332. doi: 10.11646/zootaxa.3722.3.2
Scolaro J. A., Pincheira-Donoso D. (2010). Lizards at the end of the world: Two new species of Phymaturus of the patagonicus clade (Squamata, Liolaemidae) revealed in southern Patagonia of Argentina. Zootaxa 2393, 17–32. doi: 10.11646/zootaxa.2393.1
Tennis P. S., Koonce J. F., Teraguchi M. (1979). Studies on food size as a selection pressure on body size. I. Effects of food size on fitness of two size strains of acheta domesticus L. Evolution 33, 95–103. doi: 10.2307/2407368
Toyama K. S., Junes K., Ruiz J., Mendoza A., Pérez J. M. (2018). Ontogenetic changes in the diet and head morphology of an omnivorous Tropidurid lizard (Microlophus thoracicus). Zoology 129, 45–53. doi: 10.1016/j.zool.2018.06.004
Uetz P., Freed P., Hošek J. (2023). The reptile database. Available at: http://www.reptile-database.org [Accessed March 2023].
Van Damme R. (1999). Evolution of herbivory in lacertid lizards: effects of insularity and body size. J. Herpetology 33, 663–674. doi: 10.2307/1565584
Winne C. T., Willson J. D., Gibbons J. W. (2010). Drought survival and reproduction impose contrasting selection pressures on maximum body size and sexual size dimorphism in a snake, Seminatrix pygaea. Oecologia 162, 913–922. doi: 10.1007/s00442-009-1513-8
Keywords: Liolaemidae, evolutionary optimum, diet diversification, ancestral reconstruction, Ornstein–Uhlenbeck models
Citation: Ocampo M, Pincheira-Donoso D and Rios RS (2024) Patterns of morphological diversification are influenced by dietary evolution in a highly species-rich lizard radiation. Front. Ecol. Evol. 12:1361799. doi: 10.3389/fevo.2024.1361799
Received: 26 December 2023; Accepted: 18 March 2024;
Published: 04 April 2024.
Edited by:
István Scheuring, Centre for Ecological Research, HungaryReviewed by:
Balázs Vági, University of Debrecen, HungaryCarmela Serio, University of Naples Federico II, Italy
Copyright © 2024 Ocampo, Pincheira-Donoso and Rios. This is an open-access article distributed under the terms of the Creative Commons Attribution License (CC BY). The use, distribution or reproduction in other forums is permitted, provided the original author(s) and the copyright owner(s) are credited and that the original publication in this journal is cited, in accordance with accepted academic practice. No use, distribution or reproduction is permitted which does not comply with these terms.
*Correspondence: Mauricio Ocampo, mauiocampo@gmail.com