Short-term growth of octocorals Swiftia exserta and Muricea pendula in a mesocosm
- 1Consolidated Safety Services (CSS) Inc., under contract to National Oceanic and Atmospheric Administration (NOAA), National Ocean Service, National Centers for Coastal Ocean Science, Charleston, SC, United States
- 2National Oceanic and Atmospheric Administration (NOAA), National Ocean Service, National Centers for Coastal Ocean Science, Charleston, SC, United States
To optimize fragmentation approaches for restoration applications, this study assessed fragmentation size influences on health and growth rates of mesophotic octocorals. Growth rates for Swiftia exserta (n=6) and Muricea pendula (n=9) were determined using photogrammetry in a mesocosm over a 90-day trial. No significant difference was found in growth between fragmented size classes (3, 6, and 12 cm) (S. exserta p=0.156, M. pendula p=0.393). There was a significant difference (p=0.013) in growth rates between species, with faster annual growth (mean ± SE) for S. exserta (1.490 ± 0.399 cm/year) than for M. pendula (0.550 ± 0.228 cm/year). Fragments from healthy source colonies remained healthy, while fragments from an unhealthy colony experienced tissue loss. Healthy colonies may successfully be used for fragmentation in laboratory propagation, though optimal fragment size for restoration remains debatable. Use of laboratory-based propagation techniques to recover injured species may require better understanding of factors influencing growth.
1 Introduction
Mesophotic coral ecosystems are under increasing anthropogenic pressure worldwide (Sampaio et al., 2012; Etnoyer et al., 2016; Rocha et al., 2018; Pyle and Copus, 2019). In the framework of ecosystem restoration, determining growth rates and fragmentation potential of mesophotic corals can help in assessing their suitability for outplanting. Swiftia exserta and Muricea pendula are among the most ubiquitous corals within the Northern Gulf of Mexico at mesophotic depths (Etnoyer and Cairns, 2017). These corals are characterized by their arborescent branching structures that permit access to resources in the water column and can provide shelter, feeding areas, and nurseries for many commercially important fish species (Lasker et al., 2003). Mesophotic octocorals are presumably slow growing and long lived, however empirical data using direct growth measurements are lacking.
Gorgonian corals are often overlooked in habitat restoration activities despite their contributions to the structural complexity of benthic habitats. The technique of micro-fragmentation—creating 1 cm2 fragments— has become the leading technique in accelerating the growth rate in shallow-water, reef-forming massive stony corals such as Montastraea cavernosa in the Florida Keys (Page et al., 2018). This technique allows for the propagation of corals using minimal wild livestock to replenish damaged shallow reefs. However, the influence of fragment size on the growth of gorgonian octocorals remains understudied. Assessing the potential of size-dependent growth rates can better inform restoration techniques in mesophotic coral ecosystems.
The objective of this study was to determine if fragmentation is a viable restoration tool for mesophotic coral ecosystems and whether there is an optimal fragment size for efficient laboratory-based growth. Specimens of S. exserta and M. pendula were collected and studied for 90 days under laboratory conditions using photogrammetry to assess the effects of fragmentation of various size classes on the overall health and total linear extension of the colony. The current study provides the first comparison of health and growth rates across varying size classes of fragments in live mesophotic gorgonian octocorals.
2 Methods
2.1 Sample collection and maintenance
Samples of M. pendula and S. exserta were collected from 50–65 m depths from the Northern Gulf of Mexico in October 2021 via a remotely operated vehicle (ROV)—ROV Mohawk (UNCW). Samples were collected from a distal portion of a large source colony (mean height ± SE = 0.51 ± 0.07 m) using a five-function manipulator arm (ECA Hytec ARM Micro 5E). Live samples were shipped in insulated containers at 18–22°C within 48 hours of disembarking to Hollings Marine Laboratory in Charleston, SC, and maintained in a 200-gal mesocosm. The system was supplied with artificial seawater (Red Sea Coral Pro) that mimicked in situ conditions. Water temperature was maintained at 19–21°C. The pH and salinity were recorded daily, while ammonia, nitrite, nitrate, calcium, and phosphate levels were recorded weekly. Corals were mounted to aragonite Aquadome (ReefSmart) bases and positioned upright, orientated perpendicular to the direction of flow to emulate their posture in their natural habitat. Each colony’s polyps were target-fed 5 mL from a mixture of 400 mL of seawater with 1 cube of frozen baby Artemia spp., 1 cube of frozen Calanus spp., and 1 tsp. of liquid amino acids (Polyp Lab®, USA) once daily.
2.2 Fragmentation
Coral fragments were divided into three size classes based on total linear extension (TLE): 3, 6, and 12 cm. To minimize source colony tissue loss, ImageJ (Schneider et al., 2012) was used to identify specific areas for fragmentation that resulted in less than 20% of total tissue removed from the parent colony. All fragments maintained similar characteristics and had little to no modularity (i.e. homologous branching units). For both S. exserta and M. pendula, three different colonies were used for fragmentation. Three fragments, one from each size class, were taken from distal portions of each colony, resulting in nine fragments of both species. The sample size is limited due to the increased effort required with increasing depth (Pyle and Copus, 2019). It is both difficult and expensive to collect mesophotic organisms, and therefore, small sample sizes were used for this pilot study.
Gorgonian corals are recognized for their flexible proteinaceous skeleton enclosed by soft tissue. The lack of rigid skeletons facilitates the cutting process but requires a more intensive attachment process in comparison to other species (Rinkevich, 2000). Medical-grade scissors were used to cut 3–12 cm fragments. Coenenchyme was removed from the base and the skeleton was wiped dry to encourage attachment (Barton et al., 2017; Orejas et al., 2019). A 3.175 mm hole was drilled through the middle of Aquadome Frag Mounts (ReefSmart®) to provide additional support for the coral fragments. The specimens were then individually attached to the prelabeled bases using coral glue (Elements©).
2.3 Photogrammetry and health assessments
The TLE is the summation of all the branch lengths (Lasker et al., 2003). Gorgonian corals are well suited for these measurements because they are flabellate—two-dimensional species with dichotomous branching. Photogrammetry measurements of the TLE were taken using a Canon EOS RP DSLR camera with an RF 85 mm F2 MACRO lens attachment and ImageJ software. Fragments were placed on an octagonal platform an equal distance away from the glass and perpendicular to the camera. The camera was attached to a tripod at a fixed location in front of the tank. Fragments were photographed biweekly with a colored scale-bar placed directly next to them as a reference for ImageJ analysis. Each measurement was taken three times to determine the standard error in measurement and to calculate the growth rate. The growth rate was defined as the change in TLE per 90 days (cm/90 days).
Coral fragment health was assessed weekly. Health scores for S. exserta and M. pendula were developed based on the methods of DeLeo et al. (2016) and Gugliotti et al. (2019) and modified to include reporting of additional qualitative phenotypic stress responses. The health scores were assigned to each fragment based upon the tissue and polyp activity.
Health scores were assigned as follows:
5: 75–100% tissue-covered skeleton with active polyps
4: 50%–75% tissue-covered skeleton with mostly active polyps (<50%)
3: ~50% tissue-covered skeleton with reduced polyp activity (>50%)
2: 25–50% tissue-covered skeleton with inactive polyps
1: 0–25% tissue-covered skeleton with no polyp activity
0: Bare skeleton with no polyps
Source colonies of the fragments were assessed for baseline health prior to fragmentation. All colonies began with a health score of 5 except for one colony of S. exserta that began with a health score of 2; this colony’s fragments survived 9 weeks of the 13-week study.
3 Results and discussion
All fragments originating from healthy source colonies maintained a health score of 5. The three fragments from the unhealthy colony of S. exserta did not survive in response to fragmentation and were not included in the analysis of growth (Table 1). These results indicate that fragmentation is a viable technique to increase the number of propagules if the source colony is healthy and could aid laboratory-based propagation if gender ratios are skewed. The technique of pruning vigor—a regenerative response to fragmentation (Lirman et al., 2014)—was not observed in this trial. Previous anecdotal observations of successful pruning vigor were observed within the lab, prompting the inclusion of an unhealthy source colony in the study.
Individuals exhibited a slow growth rate over the course of the study. When comparing different size class growth rates, there was no significant difference among groups (Kruskal-Wallis, p=0.156 for S. exserta, and p=0.393 for M. pendula) suggesting no effect of fragmentation size on growth rate for either species (Table 1). This finding could be attributed to the sampled location of the initial in situ specimens— the distal region of the colony. Lasker et al. (2003) indicated that gorgonian growth may be tied to modularity of the source colony, with the greatest growth rate at branch origin. Growth rates were slowest on larger colonies (Lasker et al., 2003). All samples in this study were taken from colonies of similar size and modularity (Figure 1); therefore, it would be expected that each sample would experience similar growth rates due to its origin. The fastest-growing individual was a 12 cm fragment from S. exserta that was from the smallest in situ colony (PS22-08-027), supporting previous research by Lasker et al. (2003). Hypotheses suggesting that smaller fragments elicit faster growth rates (Page et al., 2018) may not be applicable to branched soft corals, based upon the results of this study. However, future research involving fragmentation from different-sized in situ source colonies could produce significantly different growth rates.
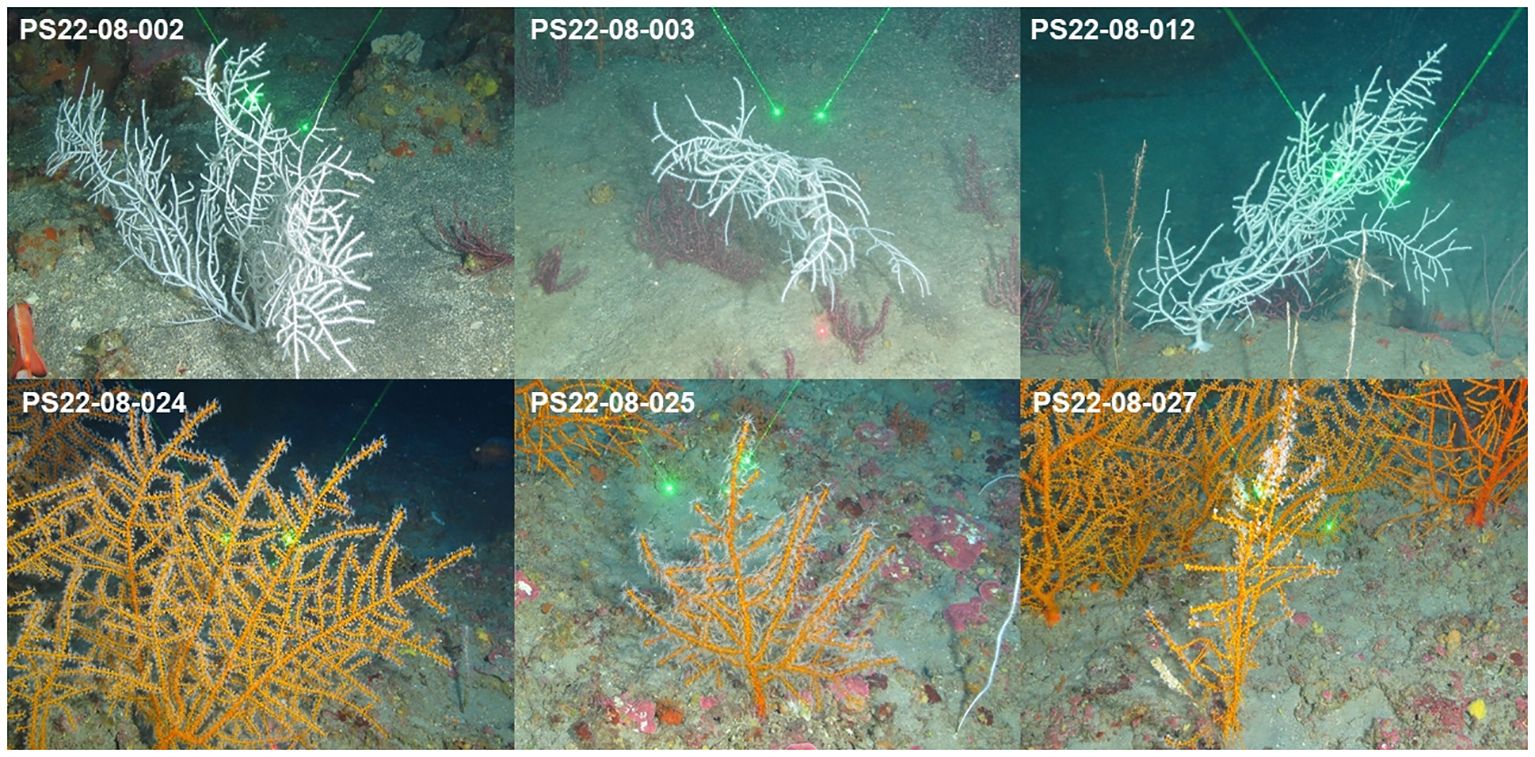
Figure 1 The three in situ colonies of M. pendula (top) and S. exserta (bottom) sampled for this study displayed similar size (height=0.51 +/- 0.07 m) and modularity. Lasers are 10 cm apart.
There was a significant difference in growth rates between the two species (Kruskal-Wallis, p=0.013). M. pendula exhibited a mean ( ± SE) growth rate of 0.136 ± 0.076 cm per 90 days, while S. exserta grew three times faster (0.368 ± 0.133 cm per 90 days; Table 2; Figure 2). When extrapolated to annual growth rates, fragments of S. exserta and M. pendula would grow an average of 1.490 ± 0.399 cm and 0.550 ± 0.228 cm, respectively, in a mesocosm over the course of a year. The growth estimates for S. exserta are similar to previously published ex situ data on shallow octocorals. Branches of Briareum asbestinum were found to grow about 2.02 cm yearly (Brazeau and Lasker, 1992) and branches of Muricea fruticosa were found to grow about 1.69 cm yearly (Grigg, 1974). Physiology may explain the difference in growth rates between the two species in the current study, but there are no published data on the feeding methods or dietary requirements for either species. Therefore, future diet experiments are needed to optimize the metabolic budget for growth of both species ex situ.
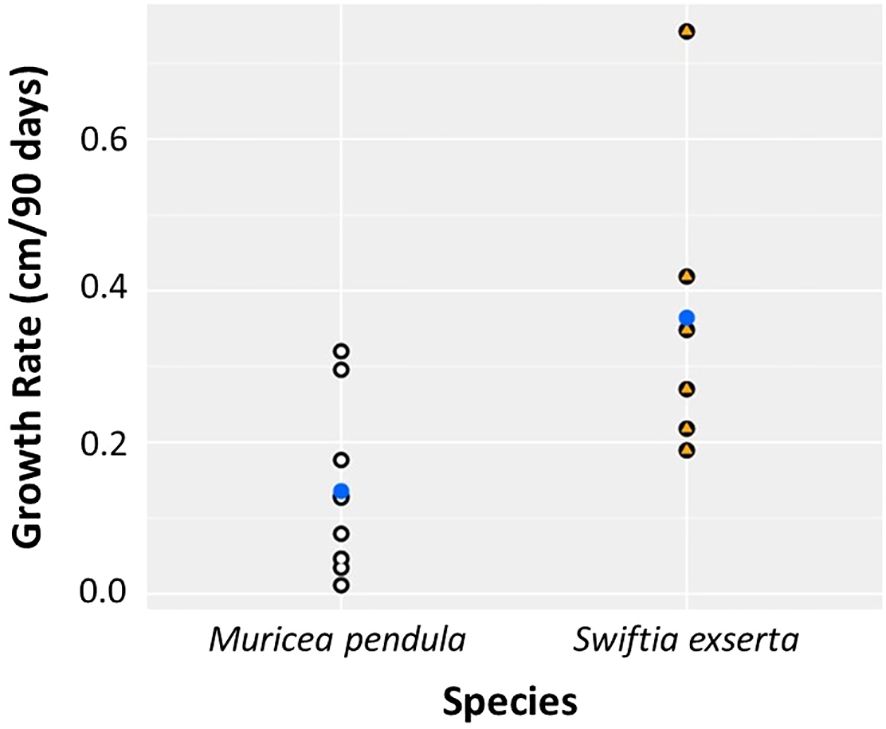
Figure 2 Growth rate differs significantly between the two species of mesophotic gorgonians, S. exserta and M. pendula, over 90 days. Points represent individuals of M. pendula (white) and S. exserta (orange). Blue points indicate the mean.
The present study provides evidence of fragment size independent growth rates, consistent with previous literature, emphasizing the role of modularity and source colony age in gorgonian growth rates (Lasker et al., 2003). Growth rates may slow as corals age, reaching an asymptote at some determinate maximum size. Specimens here were sampled from larger and presumably older colonies to minimize impacts. Future research a) that examines the growth rates of similarly sized fragments from in situ source colonies that vary in size and b) evaluates the growth rates of whole colonies ex situ and in situ will provide valuable data on the effect of fragmentation on the growth responses of gorgonians. In addition, growth rates were obtained using image-based assessment. The calculated measurement error per image was low (0.017 cm). However, their soft skeleton makes imaging difficult due to the likelihood that samples will need to be repositioned between measurements. Future studies could alleviate this problem by increasing the sample size and duration of the study to account for outliers due to photogrammetry errors.
Survivorship and growth rate of fragments are critical, but reproduction is also important, as the ultimate goal of outplanted fragments is to support wild populations. Although information about coral reproduction is imperative to restoration efforts, basic reproductive ecology remains undescribed in most mesophotic coral species (Kahng et al., 2011; Sclesinger and Loya, 2019). The effect of fragmentation on reproduction in S. exserta and M. pendula is important to consider. In the scleractinian Acropora formosa, reproduction was negatively impacted by fragmentation, as oocytes in small fragments were resorbed, presumably to satisfy the energetic requirements of growth (Okubo et al., 2007). However, Rapuano et al. (2023) demonstrated that coral fragments from reproductively mature colonies of five species of branching scleractinians retained their reproductive status even when fragmented to sub-mature sizes. This suggests that reproductive capacity is unrelated to colony size once the ontogenetic milestone of puberty is reached. Thus, comparisons of the reproductive status of fragments and mature colonies would be useful.
On mesophotic reefs in northeastern Gulf of Mexico, S. exserta and M. pendula are in the large sea fan group (>20 cm in mean height) with Placogorgia spp. and Thesea nivea. Large sea fans on reefs in this region range from 20 cm to 1 m, and occur at densities of 48 corals per 100 m2 (Etnoyer et al., 2016). The size class structure and implications of outplanting fragments on the existing community structure must also be considered. Outplanting shallow-water scleractinian Pocillopora verrucosa led to an increase in fish and coral abundance, richness, and diversity, suggesting a positive ecological influence of active restoration on community structure (Dehnert et al., 2023). Gorgonian corals have similar branching structure and may yield similar results. However, with limited knowledge on the interactions among mesophotic species, it is important that future work assesses ecological implications of outplanting, such as predation by corallivores and competition among species.
The primary aim of this study was to experimentally assess whether the fragmentation size of gorgonian octocorals influences fragment health or growth rate. The lack of effect on the health of individuals of both species sourced from healthy colonies indicates that fragmentation is a viable method to increase propagules in a laboratory. However, the significant difference in growth between the two species of octocorals may indicate that S. exserta is better suited for active restoration activities than M. pendula. The slow growth rate of both species overall suggests that these corals may not easily recuperate from disturbances in a laboratory setting. Thus, future research determining the key factors with the strongest effect on growth rate will enable optimization of fragmentation as a tool for promoting healthy stock in restored habitats.
4 Nomenclature
4.1 Resource identification initiative
ImageJ (RRID : SCR_003070)
R Project for Statistical Computing (RRID : SCR_001905)
4.2 Life science identifiers
Swiftia exserta and Muricea pendula do not have ZooBank registered life science identifiers.
Data availability statement
The raw data supporting the conclusions of this article will be made available by the authors, without undue reservation.
Ethics statement
The manuscript presents research on animals that do not require ethical approval for their study.
Author contributions
KL: Writing – original draft, Formal analysis, Investigation, Methodology. PE: Writing – review & editing, Conceptualization, Funding acquisition, Resources.
Funding
The author(s) declare financial support was received for the research, authorship, and/or publication of this article. This work was supported by the Coral Propagation Technique Development (CPT) project, one of four projects selected for the restoration of Mesophotic and Deep Benthic Communities (MDBC) under the Deepwater Horizon Oil Spill Natural Resource Damage Assessment, Open Ocean Trustee Implementation Group Final Restoration Plan 2. More information about the activities of the DWH Open Ocean Trustee Implementation Group can be found online here: https://www.gulfspillrestoration.noaa.gov/restoration-areas/open-ocean.
Acknowledgments
The authors are grateful for the support of this project by all the scientists working on the CPT project team. Thanks to committee members Dr. Jody Beers, Dr. Heather Spalding, and Dr. Mike Arendt for their valuable guidance and recommendations in scientific writing as a part of my M.S. degree. Thanks to all the individuals who have helped along the way, including the crew and science team on the R/V Point Sur and the pilots of the ROV Mohawk. Thanks also to Enrique Salgado and Arielle Pollock of the Deep-Sea Coral Ecology Lab at the National Oceanic and Atmospheric Administration’s (NOAA) Hollings Marine Laboratory (HML) in Charleston, SC, for their assistance in this project. This is Contribution No. 593. of the Grice Marine Laboratory, College of Charleston, Charleston, South Carolina.
Conflict of interest
Author KL was employed by the company CSS Inc.
The remaining author declares that the research was conducted in the absence of any commercial or financial relationships that could be construed as a potential conflict of interest.
Publisher’s note
All claims expressed in this article are solely those of the authors and do not necessarily represent those of their affiliated organizations, or those of the publisher, the editors and the reviewers. Any product that may be evaluated in this article, or claim that may be made by its manufacturer, is not guaranteed or endorsed by the publisher.
References
Barton J. A., Willis B. L., Hutson K. S. (2017). Coral propagation: a review of techniques for ornamental trade and reef restoration. Rev. Aquaculture 9, 238–256. doi: 10.1111/raq.12135
Brazeau D., Lasker H. (1992). Growth rates and growth strategy in clonal marine invertebrate, the Caribbean octocoral Briareum asbestinum. Biol. Bull. 183, 269–277. doi: 10.2307/1542214
Dehnert I., Galli P., Montano S. (2023). Ecological impacts of coral gardening outplanting in the Maldives. Restor. Ecol. 31, e13783. doi: 10.1111/rec.13783
DeLeo D. M., Ruiz-Ramos D. V., Baums I. B., Cordes E. E. (2016). Response of deep-water corals to oil and chemical dispersant exposure. Deep Sea Res. Part II 129, 137–147. doi: 10.1016/j.dsr2.2015.02.028
Etnoyer P. J., Cairns S. D. (2017). Deep-sea coral taxa in the U.S. Gulf of Mexico: depth and geographical distribution (Silver Spring, MD: NOAA). Available at: https://deepseacoraldata.noaa.gov/.
Etnoyer P. J., Wickes L. N., Silva M., Dubick J. D., Balthis L., Salgado E., et al. (2016). Decline in condition of gorgonian octocorals on mesophotic reefs in the northern Gulf of Mexico: before and after the Deepwater Horizon oil spill. Coral Reefs 35, 77–90. doi: 10.1007/s00338-015-1363-2
Grigg R. W. (1974). Growth rings: annual periodicity in two gorgonian corals. Ecology 55, 876–881. doi: 10.2307/1934424
Gugliotti E., DeLorenzo M. E., Etnoyer P. J. (2019). Depth-dependent temperature variability in the Southern California bight with implications for the cold-water gorgonian octocoral Adelogorgia phyllosclera. J. Exp. Mar. Biol. Ecol. 514-515, 118–126. doi: 10.1016/j.jembe.2019.03.010
Kahng S., Yuhuda B., Lasker H. (2011). Sexual reproduction in octocorals. Mar. Ecol. Prog. 443, 265–283. doi: 10.3354/meps09414
Lasker H. R., Boller M. L., Castanaro J., Saínchez J. A. (2003). Determinate growth and modularity in a gorgonian octocoral. Biol. Bull. 205, 319–330. doi: 10.2307/1543295
Lirman D., Schopmeyer S., Galvan V., Drury C., Baker A. C., Baums I. B. (2014). Growth dynamics of the threatened Caribbean staghorn coral Acropora cervicornis: Influence of host genotype, symbiont identity, colony size, and environmental setting. PloS One 9, e107253–e107253. doi: 10.1371/journal.pone.0107253
Okubo N., Motokawa T., Omori M. (2007). When fragmented coral spawn? Effect of size and timing on survivorship and fecundity of fragmentation in Acropora Formosa. Mar. Biol. 151, 353–367. doi: 10.1007/s00227-006-0490-2
Orejas C., Taviani M., Ambroso S., Andreou V., Bilan M., Bo M., et al. (2019). “38 cold-water coral in aquaria: advances and challenges. A focus on the Mediterranean,” in Mediterranean cold-water corals: past, present and future, Coral Reefs of the World. Eds. Orejas C., Jiménez C. (Springer, Cham), 435–471. doi: 10.1007/978-3-319-91608-8_38
Page C. A., Muller E. M., Vaughan D. E. (2018). Microfragmenting for the successful restoration of slow growing massive corals. Ecol. Eng. 123, 86–94. doi: 10.1016/j.ecoleng.2018.08.017
Pyle R. L., Copus J. M. (2019). “Mesophotic coral ecosystems: introduction and overview,” in Mesophotic coral ecosystems, Coral Reefs of the World. Eds. Loya Y., Puglise K., Bridge T. (Springer, Cham), 3–27. doi: 10.1007/978-3-319-92735-0_1
Rapuano H., Shlesinger T., Roth L., Bronstein O., Loya Y. (2023). Coming of age: annual onset of coral reproduction is determined by age rather than size. iScience 26 (5), 106533–106533. doi: 10.1016/j.isci.2023.106533
Rinkevich B. (2000). Steps towards the evaluation of coral reef restoration by using small branch fragments. Mar. Biol. 136, 807–812. doi: 10.1007/s002270000293
Rocha L. A., Pinheiro H. T., Shepherd B., Papastamatiou Y. P., Luiz O. J., Pyle R. L., et al. (2018). Mesophotic coral ecosystems are threatened and ecologically distinct from shallow water reefs. Science 361, 281–284. doi: 10.1126/science.aaq1614
Sampaio I., Braga-Henriques A., Pham C., Ocaña O., de Matos V., Morato T., et al. (2012). Cold-water corals landed by bottom longline fisheries in the Azores (north-eastern Atlantic). J. Mar. Biol. Assoc. UK 92, 1547–1555. doi: 10.1017/S0025315412000045
Schneider C. A., Rasband W. S., Eliceiri K. W. (2012). NIH Image to ImageJ: 25 years of image analysis. Nat. Methods 9, 671–675. doi: 10.1038/nmeth.2089
Keywords: growth rate, mesophotic zone, octocorals, gorgonian, fragmentation
Citation: Lange K and Etnoyer P (2024) Short-term growth of octocorals Swiftia exserta and Muricea pendula in a mesocosm. Front. Mar. Sci. 11:1390702. doi: 10.3389/fmars.2024.1390702
Received: 23 February 2024; Accepted: 25 March 2024;
Published: 09 April 2024.
Edited by:
Cliff Ross, University of North Florida, United StatesReviewed by:
Keir Macartney, The University of Texas Rio Grande Valley, United StatesYehuda Benayahu, Tel Aviv University, Israel
Copyright © 2024 Lange and Etnoyer. This is an open-access article distributed under the terms of the Creative Commons Attribution License (CC BY). The use, distribution or reproduction in other forums is permitted, provided the original author(s) and the copyright owner(s) are credited and that the original publication in this journal is cited, in accordance with accepted academic practice. No use, distribution or reproduction is permitted which does not comply with these terms.
*Correspondence: Kassidy Lange, Kassidy.lange@noaa.gov