- 1Shanghai Key Laboratory of Plant Functional Genomics and Resources, Shanghai Chenshan Botanical Garden, Shanghai, China
- 2National Key Laboratory of Plant Molecular Genetics (NKLPMG), Chinese Academy of Sciences (CAS) Center for Excellence in Molecular Plant Sciences, Shanghai, China
- 3College of Forestry, Fujian Agriculture and Forestry University, Fuzhou, China
Bretschneidera sinensis is a monotypic species of rare and tertiary relic trees mainly distributed in China. B. sinensis is a potentially valuable horticultural plant, which has significant ornamental and research value, and is a crucial tool for the study of phylogeography. The artificial cultivation of B. sinensis is of great scientific value and practical significance. In this study, we developed a direct organogenesis process of B. sinensis using mature zygotic embryos as initial materials. The highest sterile germination induction (54.5%) from the mature zygotic embryo was obtained in a Murashige and Skoog (MS) medium with 2.0 mg·L−1 6-benzylaminopurine (6-BA) and 0.2 mg·L−1 α-naphthaleneacetic acid (NAA). The highest percentage of shoot regeneration (90.37%) was attained using 1.0 mg·L−1 6-BA and 0.01 mg·L−1 NAA in the MS medium. The Woody Plant Medium (WPM) had the greatest adventitious shoot elongation rate of 93.33%. The most optimized rooting rate was 88.89% in a half-strength MS medium containing 2.0 mg·L−1 indole-3-butyric acid (IBA) and 1.0 mg·L−1 NAA. The genetic fidelity of in vitro regenerated plantlets was assessed using inter-simple sequence repeats and random amplified polymorphic DNA molecular markers, confirming the genetic uniformity and stability of regenerated B. sinensis plantlets. Our research presents an effective in vitro propagation system for B. sinensis, laying the groundwork for its germplasm conservation and large-scale production while maintaining high genetic integrity.
Introduction
Bretschneidera sinensis (Hemsl) (NCBI: txid28529; 2n = 18), also known as the Bole tree, is a valuable tree species of the Akaniaceae (turnipwood) family (Montaut et al., 2015; Wang et al., 2018). Fossil evidence shows a wide distribution of B. sinensis in the Northern Hemisphere during the Late Miocene, which declined over geological time and was restricted to a narrow geographical range in the distant mountainous areas of southern China, with some dispersed individuals appearing in eastern Asian countries in modern times (Romero and Hickey, 1976). Almost all relict species in the wild are endangered and currently restricted to historical refugia (Isah, 2015). B. sinensis is tall and straight and has attractive white flowers with pink or reddish veins, making it a potential horticultural species, according to Liu et al. (2022). Its leaf appendages have a unique structure, the upper epidermis is covered with striated cuticles, the lower epidermis is covered with flower-like papillae, and the stomatal apparatus is found only on the lower epidermis. The characteristics of B. sinensis leaf appendages reflect its long-term adaptation to its environment (Tu et al., 2012), and the species also has medicinal value for arthralgia and myalgia (Liu et al., 2010). B. sinensis is a model plant for research on angiosperm phylogeny, paleogeography, and paleoclimatology, making it an important phylogeographic research tool (Liu et al., 2021).
B. sinensis is a plant species with an extremely small population, which creates an increasing need to improve conservation techniques for its preservation (Yang et al., 2020). Effective conservation efforts are essential to prevent numerous species from extinction owing to various biotic and abiotic causes leading to their endangered status (Tommasi and Scaramuzzi, 2004). B. sinensis often grows beneath broadleaved forests, and its distribution range is constrained and confined. Its growth is very slow, and the juvenile phase is long (Liu et al., 2022). It is a mycorrhizal species, and the mortality rate of annual seedlings is high in the absence of fungal development, while the natural germination rate is less than 8% in the wild (Guo et al., 2007). This gap between seed dispersal and seedling emergence results in substantial losses, exacerbated by long-term ecological environment damage, which leads to reduced fruiting and difficulties in natural renewal, and ultimately contributes to the endangered status of B. sinensis. The International Union for Conservation of Nature (IUCN) has listed B. sinensis as “Endangered” on the IUCN Red List. B. sinensis is also recognized as a Category I endangered species in China’s Key List for the Protection of Wild Plants (Zhang et al., 2022), which requires effective conservation measures. Its survival and dissemination depend on ensuring germination and seedling growth at suitable times and locations (Qi et al., 2009; Chen et al., 2020). Thus, ex situ conservation must be performed to ensure survival (Qiao et al., 2012). Effective conservation can be achieved by the application of plant tissue culture through in vitro regeneration of the species. In vitro regeneration technology has many advantages, such as high production efficiency and the ability to obtain a large number of plant seedlings within a short period of time, greatly reducing the breeding cycle. The process is stable, reliable, and controllable because it is not affected by external environmental factors. In vitro regeneration has been widely used to protect numerous endangered monogenous woody species, such as Ginkgo biloba (Tommasi and Scaramuzzi, 2004), Glyptostrobus pensilis (Yuan et al., 2023), Metasequoia glyptostroboides (Xiong et al., 2020), and Cercidiphyllum japonicum (Fu et al., 2012). However, a few studies have been conducted on B. sinensis shoot regeneration. In experiments where shoots from B. sinensis seedlings were used as explants, researchers found a very low regeneration efficiency, with only 3.2 regenerated plantlets per explant, and the entire protocol took a long time (Guo et al., 2007). At present, there is no efficient tissue culture method for B. sinensis. Therefore, species-specific in vitro regeneration systems for B. sinensis whole-plant regeneration need to be established and optimized.
Evaluating the genetic uniformity of shoots using plant tissue culture is important owing to somaclonal variations in in vitro regenerated plantlets. DNA molecular marker analysis has been used to identify intraspecific variations in plant populations (Pillai et al., 2012). Presently, it can also be used to examine somaclonal variations in in vitro propagated plantlets. Start codon targeted polymorphism (Erişen et al., 2020), inter-simple sequence repeat (ISSR), random amplified polymorphic DNA (RAPD) (Ahmed et al., 2017; Kadapatti and Murthy, 2021), simple sequence repeats (Ioannidis et al., 2022), and amplified fragment length polymorphism (Mehta et al., 2011) have been leveraged to assess the genetic stability of regeneration plantlets. ISSR and RAPD have garnered the greatest support because they are frequently employed to investigate the genetic fidelity of regenerated species. However, research into the direct organogenesis regeneration protocol using limited B. sinensis explants is still lacking. Similarly, studies assessing the genetic fidelity of tissue-cultured plantlets—especially those transferred over 10 times—using molecular markers on initially transferred explants remain scarce.
In the present study, we aimed to establish a conservation method through direct adventitious shoot organogenesis process of B. sinensis using mature zygotic embryos as initial materials. The application of this advanced in vitro regeneration technology can be employed for endangered plant species ex situ conservation. It can also facilitate the supply of plantation seedlings, which offers a basis for boosting the proliferation of B. sinensis as a new landscape tree resource. This study represents, to our knowledge, the inaugural report on a comprehensive and efficacious regeneration system that illustrates how to obtain regenerated plantlets from limited explants in B. sinensis. In addition, we analyzed the genetic fidelity of regenerated B. sinensis shoots for the first time. This method not only paves the way for the ex situ conservation of endangered species but also contributes to ecological restoration efforts and biodiversity conservation by enabling the proliferation of B. sinensis.
Materials and methods
Seed preparation
Thirty-three B. sinensis seeds randomly selected from four thriving mature plants from the BeiGang Forest Farm, county Jing’an, Yichun (114°57′32.5″E, 28°55′11.38″N) of Jiangxi province, China, were provided by Chenshan Herbarium (Figures 1A, B). The fruit pulp was removed and seeds were rinsed under tap water and distilled water, then the water was blotted with filter paper. Surface sterilization was performed with 1‰ [v/v] potassium permanganate for 3 min, 70% [v/v] ethanol for 1 min, and then 20% [v/v] sodium hypochlorite for 12 min; sterilization procedure involved rinsing three to five times using sterile water. Afterwards, the sterilized B. sinensis seeds were placed in a pre-culture Murashige and Skoog (1962) (MS) mineral solution medium with 1‰ [v/v] plant preservative mixture (Yeasen, Shanghai, China) and without plant growth regulators (PGRs). All plant media for this study contained MS vitamins and were augmented with sucrose (3%) and gelled with agar (5.0 g·L−1). The pH was adjusted to 6.0 before adding agar and autoclaving for approximately 20 min at 121°C. The B. sinensis seeds in cultures were stored in a 16/8-h cycle of light and darkness at 25 ± 2°C, and maintained at 70% relative humidity. After 3 days, the B. sinensis seeds were checked for sterility, the endosperm was removed with a scalpel, and zygotic embryos were used for the subsequent experiments.
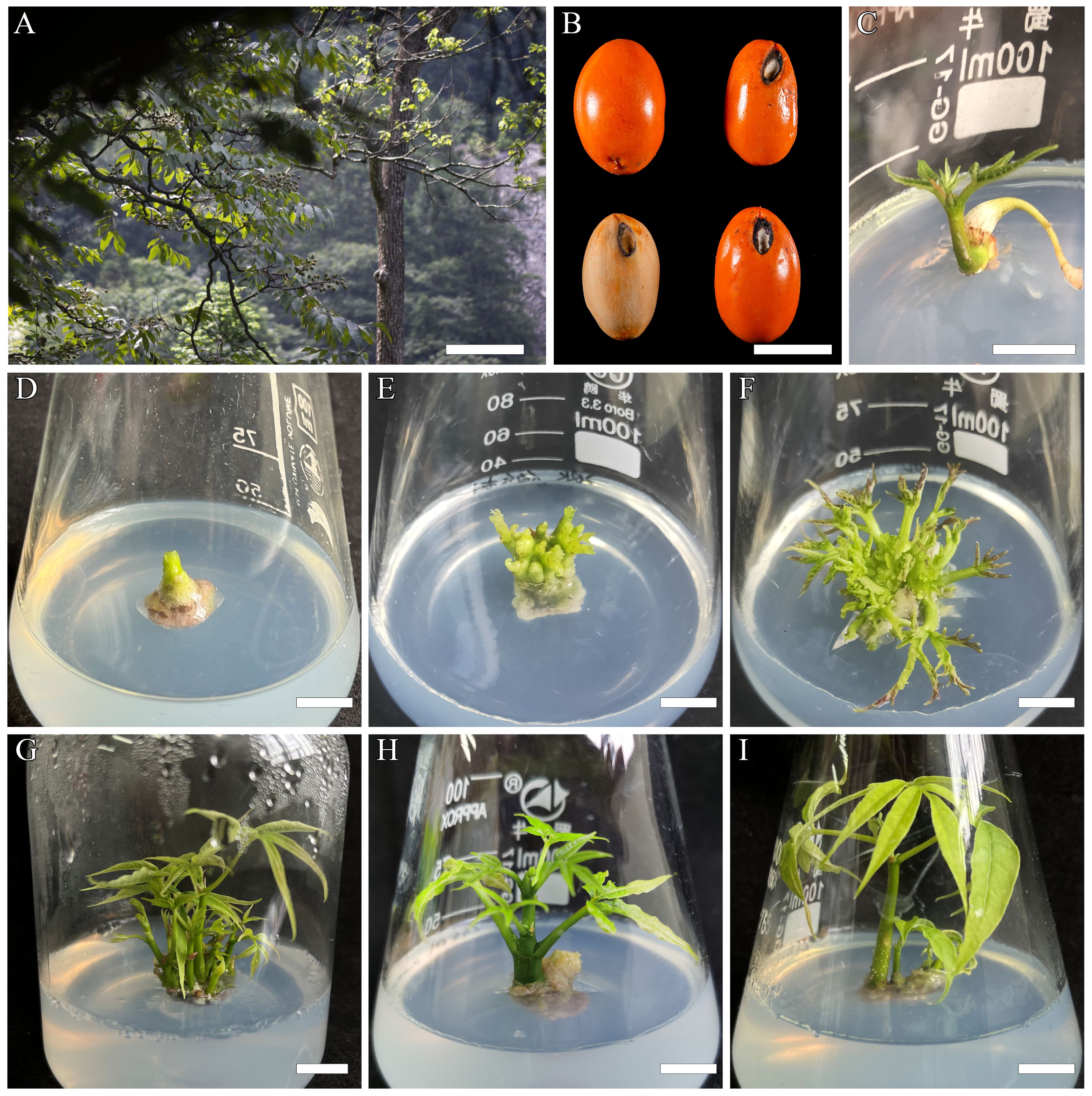
Figure 1 In vitro shoot induction and plantlets regeneration process of B. sinensis from mature zygotic embryos. (A) Adult tree of B. sinensis, bar = 33 cm. (B) Seed and fruits of B. sinensis, the bottom left is the seed with the flesh removed, bar = 1 cm. (C) Zygotic embryo germination, bar = 1.2 cm. (D) Initiation of adventitious shoots, bar = 0.9 cm. (E, F) Multiple shoots induction, bar = 0.9 cm. (G–I) Regenerated plantlets with well-developed elongation, bar in (G) = 1.12 cm, bar in (H, I) = 0.9 cm.
Germination from sterile zygotic embryos
The zygotic embryos were obtained in sterile environments and were used for inducing the zygotic embryos germination. Limited by the low fruiting rate of B. sinensis, 33 zygotic embryos were transferred in germination induction medium (GIM) in a 100-mL Erlenmeyer flask. The GIM media for this study contained MS mineral solution and vitamins, and were augmented with sucrose (3%) and gelled with agar (5.0 g·L−1). MS medium with two concentrated 6-benzylaminopurine (6-BA) (0.2 mg·L−1 and 2 mg·L−1) combined with the same amount of α-naphthaleneacetic acid (NAA) was used as the GIM for explant germination, each group had 11 zygotic embryos for the experiment, and the pH of the medium was adjusted to 6.0. With cool white fluorescent light of 60 μmol·m−2·s−1, the zygotic embryo germination was sustained in a 16/8-h cycle of light and darkness by maintaining the temperature at 25 ± 1°C and the relative humidity at 70% (Figure 1C). The germination induction percentage was recorded after 2 weeks (Table 1).
Proliferation of Shoots
The cotyledons and radicles of germinated zygotic embryos were excised and the remaining cotyledons, accounting for approximately 1–1.5 cm, were transferred to the same GIM medium until enough adventitious shoots were obtained. These shoots were used for the subsequent proliferation experiments. Afterwards, the adventitious shoots were transferred to solid MS medium added cytokinin 6-BA (0.1–3.0 mg·L−1) in combination with auxin NAA at various concentrations (0.01–1.5 mg·L−1) for shoot proliferation. The medium for proliferation was augmented with sucrose (3%), gelled with agar (5.0 g·L−1), and its pH was adjusted to 6.0 (Table 2). In a 100-mL Erlenmeyer flask, each treatment included one to three explants for the regeneration of adventitious shoots with a total of 45 replications for this experiment. With cool white fluorescent light of 60 μmol·m−2·s−1, keeping an environment temperature at 25 ± 1°C and 70% relative humidity were used to sustain the shoot proliferation cultures in a 16/8-h cycle of light and darkness. The regenerated B. sinensis shoots (Figure 1D) were sub-cultured using fresh medium (Figures 1E–G) every 2 weeks. After 8 weeks, the average shoot number and the adventitious shoot regeneration rate were counted. Subsequently, newly grown B. sinensis shoots were cut down and transferred to the shoots’ elongation medium (Figures 1H, I).
Elongation of the adventitious shoots
For the in vitro shoots’ elongation, adventitious shoots were individually dispersed and transferred to different types of basic media [MS, half-strength (1/2) MS, Woody Plant medium (WPM) (Lloyd Gregory, 1980), Douglas fir cotyledon revised medium (DCR) medium (Gupta and Durzan, 1985), double-strength (2×) DCR]. Each medium was treated with PGRs combined with 1 mg·L−1 cytokinins BA and 0.1 mg·L−1 auxin NAA, and gelled with agar (5.0 g·L−1) and the pH of the medium was adjusted to 6.0 (Table 3). Each regenerated B. sinensis shoot was transferred to a 100-mL flask, with 45 replications. The shoot elongation cultures in a 16/8-h light/dark cycle were sustained using a cool white fluorescent light of 60 μmol·m−2·s−1 at an environment temperature of 25 ± 1°C and a relative humidity of 70%. At 3-week intervals, as the B. sinensis shoots elongated, they were subcultured using new media with identical ingredients (Figure 2). After 6 weeks, the shoot elongation percentage (%) was calculated, and the elongation lengths were measured. Thereafter, regenerated B. sinensis shoots were moved to the media for rooting.
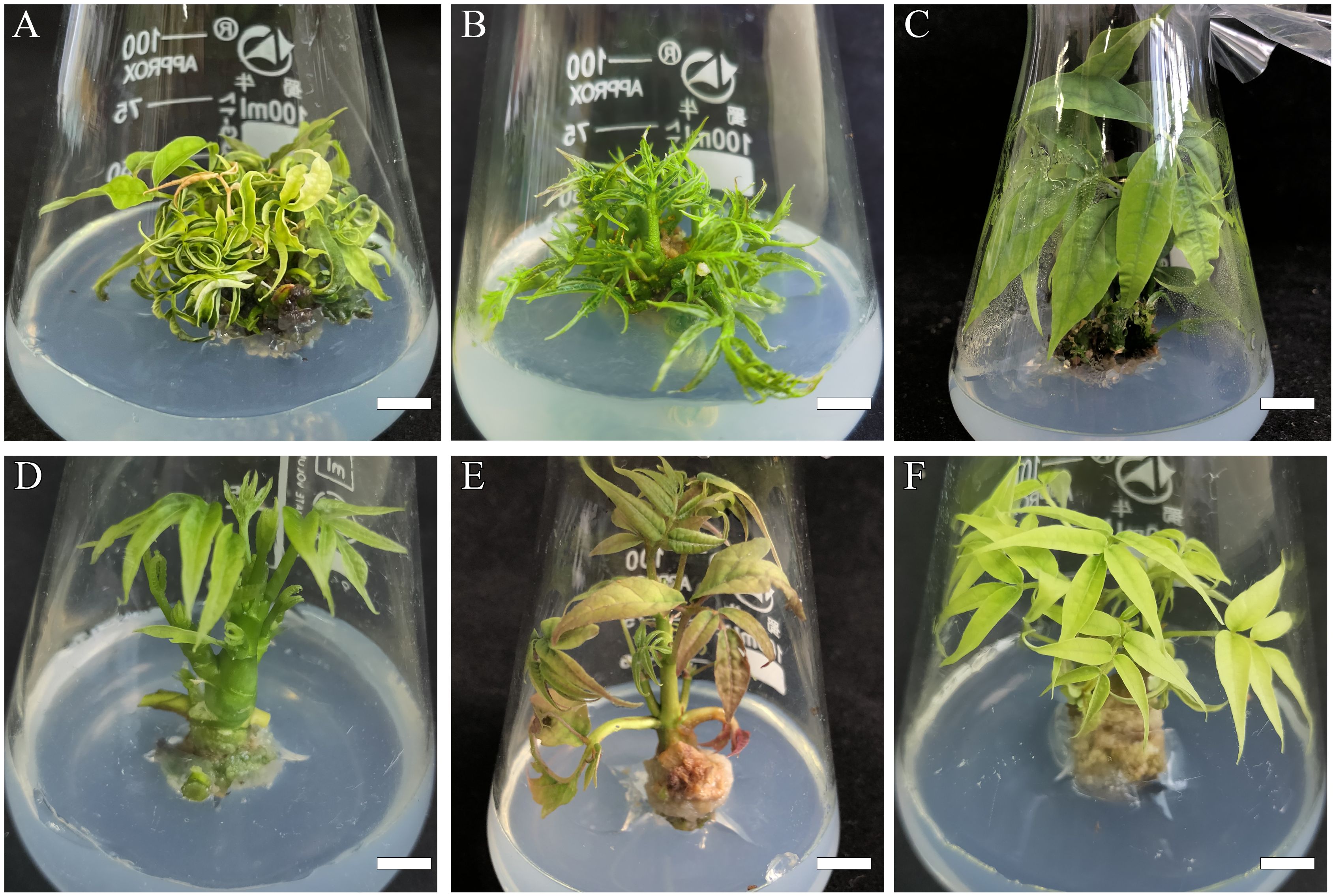
Figure 2 Effects of basic mediums on B. sinensis elongation and growth conditions. (A) Regenerated plantlets in MS basic medium, bar = 0.9 cm. (B) Regenerated plantlets in 1/2MS basic medium, bar = 0.9 cm. (C, D) Regenerated plantlets in WPM basic medium, bar in C = 0.9 cm, bar in (D) = 1.2 cm. (E) Regenerated plantlets in 2× DCR medium, bar = 1.2 cm. (F) Regenerated plantlets in DCR basic medium, bar = 0.9 cm.
Rooting of elongated shoots
For B. sinensis rooting, the elongated shoots (approximately 3–5 cm) were cut and cultured in the 1/2MS and WPM basic media (50 mL) of various strengths with auxin NAA singly or with auxin indole-3-butyric acid (IBA) in polystyrene culture vessels (125 × 110 mm). The media in the experiments all contained 30 g·L−1 sucrose, and only in the rooting experiments did some of the media reduce the sucrose content to 20 g·L−1, which is labeled in Table 4, gelled with agar (5.0 g·L−1), and the medium was adjusted to 6.0. For this experiment, there was one regenerating shoot with 45 replications. The rooting cultures in a 16/8-h cycle of light and darkness were sustained using a cool white fluorescent light of 60 μmol·m−2·s−1, and by maintaining the environment temperature of 25 ± 1°C and relative humidity of 70%. After cultivating for 10 weeks with no subculture, the rooting rate for each treatment was evaluated (Figure 3).
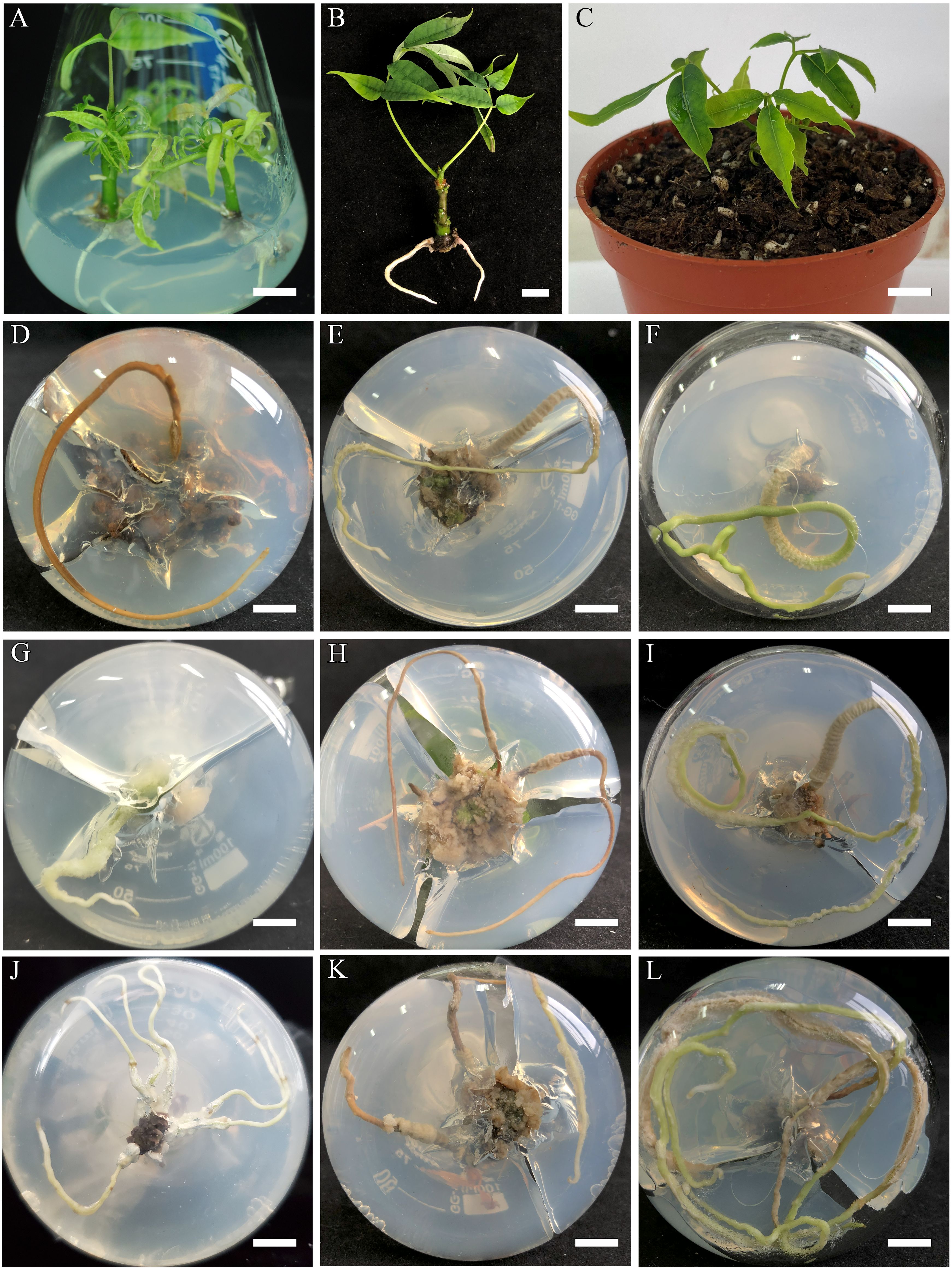
Figure 3 Rooting of B. sinensis regenerated shoots. (A) Rooting of B. sinensis regenerated shoots, bar = 0.83 cm. (B) Regeneration of B. sinensis plantlets, bar = 0.6 cm. (C) Acclimatized plants in 3 weeks, bar = 2 cm. (D) 1/2MS (sucrose-), bar = 1.18 cm. (E) 1/2MS + IBA 1 mg·L−1 + NAA 2 mg·L−1, bar = 0.83 cm. (F) 1/2MS + 0.5 mg·L−1 NAA + 1 mg·L−1 IBA, bar = 0.83 cm. (G) 1/2MS + 1 mg·L−1 NAA + 3 mg·L−1 IBA, bar = 0.83 cm. (H) 1/2MS+ 1 mg·L−1 NAA + 2 mg·L−1 IBA (sucrose-), bar = 0.83 cm. (I) 1/2MS + 1 mg·L−1 NAA + 2 mg·L−1 IBA, bar = 0.83 cm. (J) 1/2MS + 2 mg·L−1 IBA, bar = 0.83 cm. (K) 1/2MS + 2 mg·L−1 NAA + 1 mg·L−1 IBA, bar = 0.83 cm. (L) 1/2MS + 2 mg·L−1 NAA + 3 mg·L−1 IBA, bar = 0.83 cm.
Acclimatization
After rinsing the B. sinensis plantlets thoroughly under running water, the medium around the roots was washed; then, the plants were transplanted into a plastic container with a 15-cm diameter (Figure 3C); the substrate composition contained peat, organic cultivation soil, and perlite at a ratio of 3:6:1 (v/v). Transparent plastic bags were used to cover the plastic pots to preserve humidity under acclimatization at a 16/8-h light/dark cycle; the growth chambers were operated under a cool white fluorescent light of 33.73 μmol·m−2·s−1 and the environment temperature was maintained at 25 ± 1°C. After 3 weeks, as the plantlets adapted, the plastic covers were progressively taken off. Plant survival rates were assessed 6 weeks later.
Genetic fidelity analysis
One zygotic embryo germinated in medium with cytokinin 2 mg·L−1 6-BA and 0.2 mg·L−1 auxin NAA (Table 1) was selected, and the experiments were conducted with the seedling line it produced for genetic fidelity analysis. Plantlets transferred for the first time after germination were identified as the mother plant, and plantlets transferred 10 times or more were selected for experiments. Leaves from in vitro plantlets that were transferred the first time and over 10 times were collected for DNA extraction. Liquid nitrogen was used to freeze fresh leaves, and the cetyl-trimethyl ammonium bromide (CTAB) technique was used to isolate genomic DNA (Gawel and Jarret, 1991). Agarose gel electrophoresis (0.8%) was used to qualify the purity of the isolated DNA, after the extracted DNA had been added with 7 µL of RNase (100 mg·mL−1) and measured using NanoDropC (Thermo Fisher Scientific, Waltham, USA) at 260 nm and 280 nm (Figure 4A). The ISSR assay was conducted in 20 μL of reaction mixture containing 10 μL of 2×Taq PCR Mix, 0.5 mmol primers (Sangon, Shanghai, China), and 100 ng of genomic DNA. The ISSR amplification was performed in thermal cycles (Eppendorf ESP-S). The thermal conditions used for the ISSR assay were as follows: initial denaturation at 95°C for 5 min, 40 cycles at 95 for 30, 46°C for 30 s, 72°C for 130 s, and final extension for 10 min at 72°C. The RAPD amplification thermal conditions used in the study were initial denaturation at 95°C for 5 min, 40 cycles at 95°C for 30 s, 38°C for 30 s, 72°C for 130 s, and a final extension for 10 min at 72°C. Amplified products and GelRed staining were resolved in 1.2% agarose gel (1×TAE); then, the gel was documented using a gel doc system (Bio-Rad, Hercules, CA, USA) under UV light. The clear DNA bands were considered when estimating the size of the amplification products using the DL2000 DNA Ladder or the 5,000-bp DNA marker (Vazyme, Nanjing, China). Then, 29 ISSR primers and 20 RAPD primers were evaluated, producing scoreable bands, and repeatable bands were chosen for further amplification.
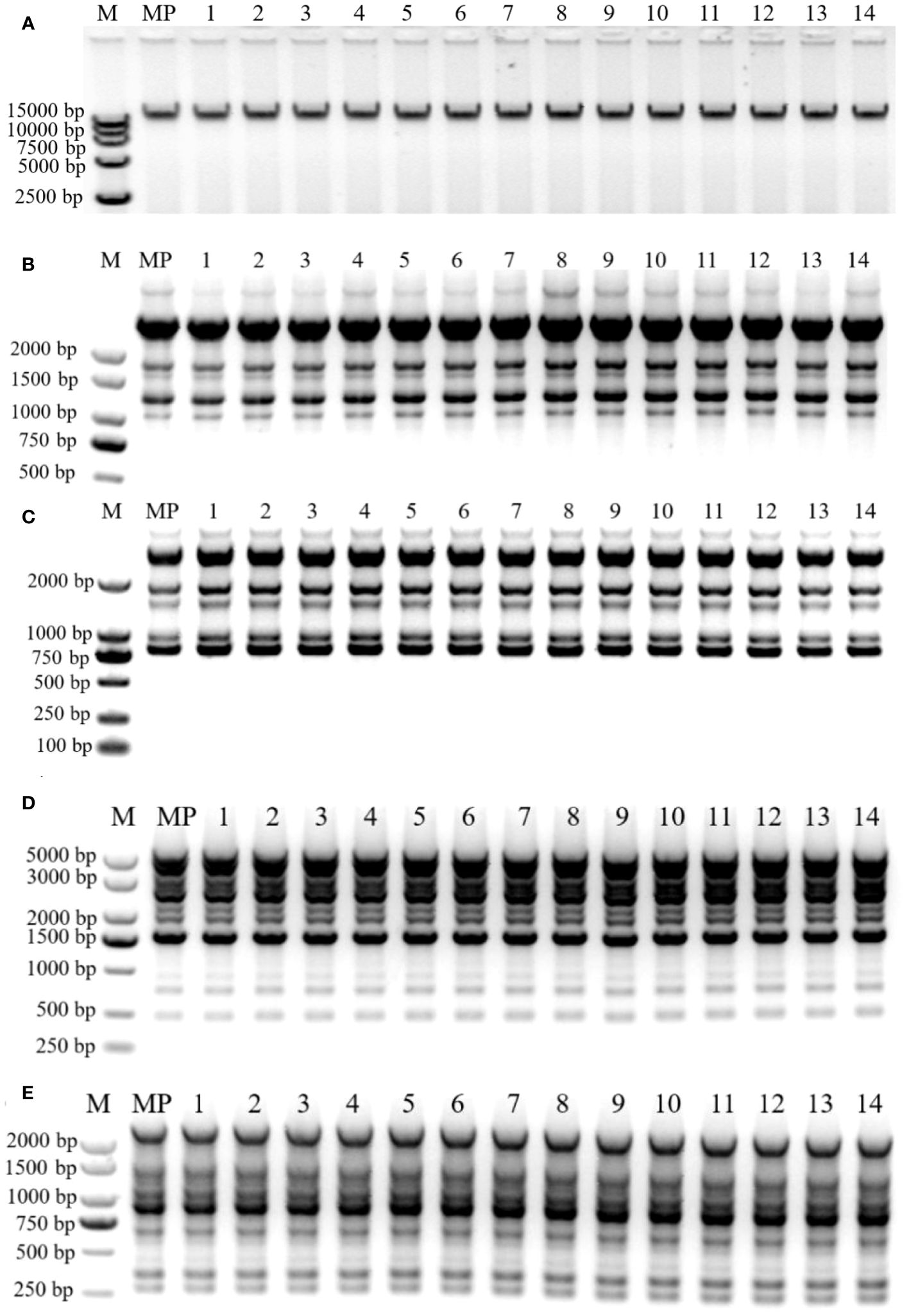
Figure 4 Genetic fidelity of regenerated plants. (A) Genomic DNA extraction electrophoresis assay of B. sinensis. (B, C) ISSR profiles generated by PCR amplification with primer UBC-874 (B) and UB-C892 (C). Lane M: Molecular marker (100 bp–5 Kb); MP: Mother plant, the first-time transferred plantlet; Lanes 1–14: Regenerated plants transferred over 10 times. (D, E) RAPD profiles generated by PCR amplification with primer S130 (D) and S144 (E). Lane M: Molecular marker (100 bp–5 Kb for S130; 100 bp–2 Kb for S144); MP: Mother plant, the first-time transferred plantlet; Lanes 1–14: Regenerated plants transferred over 10 times.
Statistical analysis
The following equations were employed to determine various in vitro regeneration parameters:
All experimental results were evaluated using the one-way ANOVA with Tukey’s post-hoc multiple comparison testing except seed germination owing to the insufficient number of explants using SPSS (IBM SPSS Statistics 27.0). At p ≤ 0.05, means were deemed statistically significant.
Results
Seed preparation and germination
After surface sterilization, 17 of the 33 B. sinensis seeds responded, while the others were contaminated and debilitated. With the seed preparation and germination, we found that the highest germination rate (54.5%) was achieved in medium supplemented with 2 mg·L−1 6-BA and 0.2 mg·L−1 NAA (Figure 1C), followed by 36.3% in the medium with equal amounts of 2 mg·L−1 6-BA and 2 mg·L−1 NAA. The germination rate was 27.2% in the medium containing 0.2 mg·L−1 6-BA and 2 mg·L−1 NAA, which had the same rate as that of callus induction (Table 1).
Shoot induction and proliferation
For the induction and proliferation of the B. sinensis adventitious shoot, when the ratio of cytokinins 6-BA/auxin NAA was 10/1 in the MS media, and the concentration of the two PGRs were 1.0 and 0.1 mg·L−1, respectively, 90.37 ± 1.96% of explants regenerated new shoots. Additionally, 16 ± 0.29 shoots formed per explant, which was the highest induction effect. This was followed by the medium with the same concentration of 6-BA and an increased NAA (0.3 mg·L−1), which had a shoot regeneration of 84.44 ± 5.88% and a low shoot number per explant of 7 ± 0.10 (Table 2). Therefore, the shoot regeneration rate and adventitious shoot number per explant induced by the different formulations were inconsistent and disproportionate (Table 2). Both shoot regeneration rates were high in a medium containing 6-BA (1 mg·L−1) combined with NAA (0.05 to 0.3 mg·L−1). Culture media enriched with 6-BA and NAA at a ratio of 10/1 or lower generally had more adventitious shoots on average.
However, there were certain exceptions. Regardless of whether the ratio of cytokinin 6-BA and auxin NAA is 10/1 or lower than 10/1, a certain concentration must be achieved for some effect. In media containing 0.1 mg·L−1 6-BA and 0.01 mg·L−1 NAA, as well as 0.3 mg·L−1 6-BA and 0.03 mg·L−1 NAA, both the induction rate and number of adventitious shoots were very low. In addition, when the ratio of 6-BA and auxin was 2/1–3/1, the average number of adventitious shoots in these media was very low, no more than six, and no lower than two (Table 2), which is unfavorable for the proliferation of adventitious shoots.
Effects of the basic medium on elongation and growth status
Before elongation induction, the plantlets were sub-cultured in a fresh shoot regeneration medium for 4 weeks. We used the cytokinin BA/auxin NAA combination with 1/0.1 mg·L−1 in five types of different basic media (MS, 1/2MS, WPM, 2×DCR, and DCR) with different nutrient levels for adventitious shoot elongation. The WPM basic medium yielded the optimal elongation rate of 93.33 ± 3.76% and the longest average shoot length was 5.13 ± 0.07 cm (Figures 2C, D). This was followed by the 2× DCR and DCR media, with shoot elongation percentages of 82 ± 5.86 and 71.33 ± 4.33%, respectively (Figure 2E), and average shoot lengths of 5.03 ± 0.07 cm and 4.97 ± 0.03 cm, respectively (Figure 2F), close to the average length of the WPM group. From a growth perspective, plants in the WPM group were green and robust, whereas plants in the 2× DCR and DCR groups exhibited leaf chlorosis.
In the same 1/2MS and MS media supplements, both the shoot elongation induction effect and elongated length were significantly lower (p< 0.05) than those in WPM (Table 3; Figures 2A, B). For the 1/2MS basic medium, 42.22 ± 5.88% of the shoots experienced elongation with an average elongation length of 3.13 ± 0.15 cm. For the MS group, the shoot elongation rates and lengths were considerably lower, at 17.78 ± 4.44% and 1.97 ± 0.12 cm, respectively. Small shoots with crinkle leaves were seen in the MS and 1/2MS groups, and the degree of crinkled leaves in the 1/2MS medium was lower than that in the MS medium, indicating that a suitable basic medium can favor leaf stretching and plantlet elongation. The basic medium considerably boosted both the shoot elongation average and percentage. Thus, the WPM basic medium is relatively suitable for adventitious shoot elongation in B. sinensis.
Rooting
Here, 1/2 MS medium and WPM were used for rooting induction in the basic medium, achieving good rooting (Figures 3A, B). The highest adventitious root induction rate was 88.89 ± 2.2% (Table 4; Figure 3H) in 1/2MS medium supplemented with 2.0 mg·L−1 IBA, 1.0 mg·L−1 NAA, and sucrose 20 g·L−1 (sucrose-), followed by the same component with an additional 10 g·L−1 sucrose, with an adventitious root induction rate of 80 ± 3.8% (Figure 3I). However, in the WPM medium with the same PGRs, the rate was 68.89 ± 2.2%, the highest adventitious root induction rate in the WPM group.
Compared to the other media, the adventitious root induction rates of the two treatments in the 1/2 MS medium were considerably greater (p ≤ 0.05). The combination of NAA and IBA was more effective than auxin alone for rooting. In each basic medium group, the adventitious root induction rate peaked at auxin levels of 3 mg·L−1 overall (Figures 3H, I). The group with auxin levels below 3 mg·L−1 had a low induction rate but good rooting status (Figures 3D–F, J). However, the experimental group with high auxin levels (>3 mg·L−1) had a high induction rate but poor adventitious root quality, which was brittle, and the epidermis was swollen and loose (Figure 3G, K, L). In addition, the reduction in sucrose promoted root formation to a certain extent in the 1/2 MS basic medium but did not significantly increase the rooting percentage in the WPM basic medium.
Acclimatization
Acclimatized B. sinensis plantlets exhibited a high survival rate of 72% after 6 weeks of acclimatization, and we observed the formation of a compound leaf from the simple leaf split. Plant material from the same batch of transplants grew consistently in the same medium and growth time; the adapted plantlets developed healthily and verdant green leaves, and exhibited typical morphological and growth traits of the plant species (Figure 3C).
Genetic fidelity of regenerated shoots
We used 29 ISSR and 20 RAPD primers for the genetic fidelity evaluation. Of these, 91 distinct monomorphic bands were generated using five RAPD and seven ISSR primers, which were suitable for subsequent PCR amplification reactions (Supplementary Table 1). A single ISSR primer yielded an average of six bands for the ISSR markers, with banding patterns ranging from four (UBC-853) to eight (UBC-824) (Supplementary Table 1). Altogether, 44 bands were generated using ISSR primers, ranging in size from 250 to 3,000 bp (Supplementary Table 1). Figures 4B, C show the band patterns of UBC-874 and UBC-892, respectively. The RAPD primers yielded 47 bands with an average of 9 per primer. The average number of bands created by the isolated RAPD primer ranged from 6 (S226) to 13 (S208), and the bands ranged from 200 to 3,000 bp (Supplementary Table 1). The S130 and S144 banding patterns are shown in Figures 4D, E, respectively. The monomorphic banding patterns generated by DNA molecular markers demonstrate that the mother plants and regenerates have a stable genetic makeup. From the ISSR and RAPD markers, 44 and 47 monomorphic bands were generated, respectively.
Discussion
Despite significant advancements in understanding B. sinensis through various genetic and ecological studies, practical conservation efforts are hindered by the species’ reproductive challenges. Current research on B. sinensis has mainly focused on chloroplast genomic resources, genetic divergence (Shang et al., 2022), demographic histories (Zhang et al., 2022), phylogeography, conservation genetics (Wang et al., 2018), and glucosinolate diversity (Montaut et al., 2015). Owing to the rare and endangered nature of B. sinensis, its unique pedigree, and geographical research significance, ex situ conservation and artificial expansion of this species have urgently necessitated further research. Our findings demonstrate a remarkable increase in germination rates, with over 50% of treated seeds sprouting after pulp removal—a significant improvement over natural germination rates observed in wild populations. Our study illuminates the physical dormancy mechanism inherent to B. sinensis seeds, where the seed coat and embedded chemicals impede germination, and showcases ayu novel approach to circumvent this barrier, enhancing germination success.
Owing to the lack of a well-established in vitro regeneration system, studies on the molecular mechanisms of regeneration in non-model woody plants are rarely reported, and a general belief stipulates that woody plants are more difficult than herbaceous plants for plant in vitro regeneration (Thorpe and Harry, 1990; Phillips and Garda, 2019; Abdalla et al., 2022; Nowakowska et al., 2022; Zdravković-Korać et al., 2022). Current research on the molecular mechanisms of plant in vitro regeneration is mainly focused on model plants, represented by Arabidopsis thaliana (Atta et al., 2009; Sugimoto et al., 2010; Zhai and Xu, 2021; Zhai et al., 2023). Many studies have shown that the use of PGRs exerts the most important effect on shoot proliferation during in vitro regeneration (El-Sayed et al., 2020; Fernandes et al., 2020). The cytokinin signaling pathway Arabidopsis histidine kinase-Histidine-containing phosphotransfer protein-Arabidopsis response regulator (AHK- AHP-ARR) activates the key gene WUSCHEL (WUS) for apical stem cell maintenance (Dai et al., 2017; Meng et al., 2017; Wang et al., 2017; Zhang et al., 2017), which through interaction with STM (Su et al., 2020), coordinately regulates the expression of CLV3. The WUSCHEL-SHOOT MERISTEMLESS-CLAVATA3 (WUS-STM-CLV) module ensures the morphogenesis of bud primordia. B-ARR transcription factors are key genes that activate the transformation of bud cell fate. On the one hand, B-ARR interacts with members of the CLASS III HOMEODOMAIN-LEUCINE ZIPPER (HD-ZIP III) family, directly binding to the WUS promoter to activate its transcription (Dai et al., 2017; Meng et al., 2017; Wang et al., 2017; Zhang et al., 2017), and on the other hand, it maintains the balance of endogenous auxin and cytokinin by inhibiting YUCCA (YUC)1/4 (Cheng et al., 2013; Meng et al., 2017). Additionally, cytokinins and auxins influence the expression of the aforementioned genes by regulating histone modifications and the degree of chromatin openness (Wu et al., 2022). Auxin is also a decisive factor in initiating plant regeneration, with the auxin signaling pathway regulating cell division and pluripotency through key transcription factors, including members of the LATERAL ORGAN BOUNDARIES (LBD) family LBD16/29 (Fan et al., 2012; Xu et al., 2018), GRAS family members SCR and SHR (Kim et al., 2018; Zhai et al., 2023), PLETHORA family members PLT1/2 (Kareem et al., 2015; Zhai and Xu, 2021) and PLT3/5/7 (Kareem et al., 2015; Varapparambath et al., 2022), and WUSCHEL-RELATED HOMEOBOX (WOX) family members WOX11 (Liu et al., 2018) and WOX5/7 (Kim et al., 2018; Zhai and Xu, 2021), all of which play significant roles in the establishment and maintenance of plant cell pluripotency. In plant in vitro regeneration, the ratio of exogenously added auxins and cytokinins influences the differentiation direction of regenerating seedlings. A regeneration study on Andrographis echioides demonstrated that the maximum regeneration efficiency and number of regenerations were achieved when the 6-BA/IAA ratio was 15/1 (Savitikadi et al., 2020). For Populus trichocarpa, a cytokinin/auxin ratio of 5/1 resulted in the highest bud induction rate (Ma et al., 2022). However, the specific ratios and intensities of these hormones have not been clearly determined across different plants, and varying these ratios with different hormone strengths can lead to dramatically different outcomes (Song et al., 2023). We observed both high and low induction rates at a 6-BA/NAA ratio of 3/1. The intensity of PGRs was also important; as the intensity of hormone combinations of the same ratio increased, shoot regeneration was first increased and then decreased; this phenomenon has also been reported during in vitro regeneration in other species (Shekhawat et al., 2021), indicating that both hormone combinations of ratio and intensity are important for the shoot regeneration rate (Yan et al., 2023). The in vitro regeneration of non-model plants typically relies on gradient hormone combinations or empirical experiments. Our findings pave the way for future investigations into the molecular mechanisms underpinning PGR effects on in vitro regeneration, crucial for refining micropropagation techniques across diverse plant species.
Elongation culture is a critical step in the in vitro regeneration system for B. sinensis, setting the stage for successful rooting by producing robust plantlets capable of resisting nutrient consumption by exogenous hormones. Our findings indicate that the choice of culture medium significantly impacts plantlet elongation and health, which formed different growth states and leaf colors: MS and 1/2MS media resulted in smaller adventitious shoots with curled, green leaves; WPM medium facilitated better shoot elongation and healthier green leaves, whereas DCR and 2× DCR media also supported shoot elongation but led to yellow and red leaf coloration, respectively. Studies on cultivated woody plants with obvious stems, such as Eucalyptus benthamii explant sources (Esposito-Polesi et al., 2020), Larix olgensis (Yan et al., 2023), Juniper species (Hazubska-Przybył, 2019), and Persea americana Mill. cv. Hass (Osorio et al., 2018), have achieved plantlet elongation by lowering the concentration of basic medium or the phytohormone concentration. In the present study, selecting the appropriate medium and reducing the nitrogen and phosphorus contents were conducive to adventitious shoot elongation at the stage of obvious stem differentiation (Table 1; Figure 1). A novel discovery in our study was the relationship between leaf coloration in B. sinensis and potassium ion concentration, underscoring the nuanced role of specific nutrients in plantlet development. This insight is critical for tailoring regeneration protocols to accommodate the unique physiological needs of woody plants. Woody plants have better-developed vascular tissues, and MS basic medium usually fails to yield good results in the gradual transition of large numbers of adventitious shoots to lignification. Previous anatomical studies have shown that the xylem vessel diameter of B. sinensis is small and water and inorganic salt transport is inefficient (Carlquist, 1996).
Rooting in vitro is a pivotal yet challenging step in the regeneration of woody plants, especially for recalcitrant and mycorrhizal species that exhibit unique rooting behaviors. Successful induction of rooting is essential for the regeneration of woody plants. Most of the recalcitrant species that are difficult to root are woody plants, which exhibit rooting differences. So far, many other plants are symbiotic with fungi in nature, which are difficult for tissue culture and in vitro regeneration system, and B. sinensis, which is a mycorrhizal plant, is one of them. For in vitro rooting, numerous studies favor the 1/2 MS basic medium and addition of auxin to induce rooting (Wei et al., 2017; Amiri et al., 2020). This study specifically aimed to optimize the in vitro rooting conditions for B. sinensis by comparing the efficacy of 1/2 MS and WPM media, which has lower inorganic salt concentration. A key finding of our research is that the 1/2 MS medium, with its balanced nutrient content, proved significantly more effective for B. sinensis rooting than the WPM medium. Our results underscore the critical balance between reducing inorganic salt concentration and ensuring sufficient nutrient availability for rooting, highlighting the inefficacy of solely reducing salts without considering overall nutrient needs. Normally, host plants translocate organic nutrients to mycorrhizal fungi, and mycorrhizal fungi translocate mineral nutrients to host plants (Wang et al., 2022). Under the aseptic conditions of in vitro regeneration, B. sinensis requires more nutrients for adventitious root formation, but no additional sucrose is required for root growth under aseptic conditions; therefore, the more nutrient-rich 1/2 MS with reduced sucrose would be more suitable for in vitro rooting of B. sinensis plantlets. The nuanced role of phytohormone concentrations in our study aligns with findings from research on other species, suggesting a general principle that overly high concentrations may hinder the development of a healthy root system. This insight is particularly valuable for the in vitro rooting of mycorrhizal woody plants. These results are consistent with those of research on red raspberries (Rubus idaeus) (Kim and Dai, 2020), which, despite the use of a high amount of auxin additive, does not necessarily result in good rooting conditions, as it makes the roots thick and loose, resulting in a fragile root system that is not conducive to rooting and transplanting. Similar conclusions have been reached for the cannon ball tree (Couroupita guianensis aubl.) (Shekhawat and Manokari, 2016).
Genetic fidelity studies play a crucial role in preserving the genetic integrity of plant species during the process of domestication and cultivation (Li et al., 2005). Nowadays, these studies become particularly relevant in plant tissue cultures, with the risk of somaclonal variations (Ferreira et al., 2023). This phenomenon, characterized by inconsistent traits in regenerated plantlets compared to the mother plant, presents challenges for large-scale production (Eeckhaut et al., 2020). Factors such as DNA methylation changes, oxidative stress, nutrient availability, and the use of high concentrations PGR have been identified as key contributors to these genetic and epigenetic alterations. For instance, studies on pineapple callus cultures and rice have highlighted the significant role of epigenetic modifications, such as DNA methylation changes, in driving somaclonal variations (Stroud et al., 2013; Lin et al., 2019). Moreover, oxidative stress (Bednarek and Orłowska, 2020), nutrient availability (Duarte-Aké and De-la-Peña, 2021), and the application of high PGR concentrations (Ferreira et al., 2023) may influence somaclonal variation, leading to genetic and epigenetic alterations in tissue culture plantlets. The development of stable and reliable regeneration systems becomes paramount, not only for mitigating the effects of somaclonal variation but also for enabling precise genetic studies and transformations. Such systems are essential for dissecting the genetic underpinnings of regeneration and mutation. Our protocol is controlled and reliable, as adventitious shoots transplanted more than 10 times are consistent with the genetic stability of first-generation donor material. Our findings align with similar genetic fidelity achievements in species like Cornus alba, Thunbergia coccinea Wall., Manglietiastrum sinicum, Ranunculus wallichianus, and Haplophyllum tuberculatum (Erişen et al., 2020; Srinivasan et al., 2021; Alsafran et al., 2022; Sultana et al., 2022; Luo et al., 2023), where direct organogenesis shoots exhibited consistent genetic profiles, as verified by ISSR and RAPD markers (Backiyarani et al., 2021).
One inherent limitation of our study stems from B. sinensis’s endangered status and small population, which restricted our sample size and, consequently, the scope of our preliminary research. The cherished and endangered status of B. sinensis prevents the performance of related studies. In the future, we aim to broaden our exploration of regeneration techniques by employing stems and leaves as explants, develop a comprehensive large-scale breeding system, and delve into the growth mechanisms of B. sinensis. The methodology established in this research advances the conservation of B. sinensis and sets a precedent for ex situ conservation and artificial propagation of other endangered species, providing a scalable model for biodiversity conservation, and this study also contributes valuable insights into the field of species-oriented plant tissue culture and provides a basic reference for further exploration of non-model woody plant regeneration.
Conclusions
Our study presents the first fully effective regeneration system for B. sinensis seeds, marking a significant breakthrough in the species’ conservation efforts. We meticulously investigated the impact of PGRs at varying ratios and across different basic media, establishing a methodology that ensures successful large-scale production and conservation of B. sinensis. Utilizing ISSR and RAPD markers for clonal fidelity tests, we have demonstrated the genetic fidelity of the regenerated plants, further validating our protocol’s capacity to produce high-quality B. sinensis plantlets. Our efficient and reliable protocol transcends B. sinensis conservation, offering a promising model for the rescue and ex situ conservation of a wide range of endangered species. Our groundbreaking approach not only paves the way for the efficient ex situ conservation of B. sinensis but also sets a precedent for applying innovative regeneration techniques in the conservation of other endangered species, expanding the horizons of plant conservation research.
Data availability statement
The original contributions presented in the study are included in the article/Supplementary Files. Further inquiries can be directed to the corresponding author.
Author contributions
XY: Data curation, Investigation, Methodology, Validation, Visualization, Writing – original draft, Writing – review & editing. KZ: Conceptualization, Data curation, Investigation, Methodology, Validation, Writing – original draft. PL: Resources, Validation, Writing – review & editing. XZ: Validation, Writing – review & editing. ZZ: Investigation, Resources, Validation, Writing – review & editing. HZ: Formal analysis, Methodology, Resources, Validation, Writing – review & editing. MZ: Conceptualization, Funding acquisition, Investigation, Project administration, Supervision, Writing – review & editing.
Funding
The author(s) declare that financial support was received for the research, authorship, and/or publication of this article. This work was supported by the Chenshan Special Fund for the Shanghai Landscaping Administration Bureau Program (G232409) and the National Science and Technology Major Project of China (2018ZX0802003-005-001).
Acknowledgments
The authors thank professor Zhihong Xu, academician of the Chinese Academy of Sciences, for his guidance on the research. We thank senior engineer Binjie Ge for providing B. sinensis seeds. This work was supported by the Chenshan Special Fund for the Shanghai Landscaping Administration Bureau Program (G232409), Special Fund for Scientific Research of Building National Botanical Garden (XM04-10) and the National Science and Technology Major Project of China (2018ZX0802003-005-001).
Conflict of interest
The authors declare that the research was conducted in the absence of any commercial or financial relationships that could be construed as a potential conflict of interest.
Publisher’s note
All claims expressed in this article are solely those of the authors and do not necessarily represent those of their affiliated organizations, or those of the publisher, the editors and the reviewers. Any product that may be evaluated in this article, or claim that may be made by its manufacturer, is not guaranteed or endorsed by the publisher.
Supplementary material
The Supplementary Material for this article can be found online at: https://www.frontiersin.org/articles/10.3389/fpls.2024.1259925/full#supplementary-material
References
Abdalla, N., El-Ramady, H., Seliem, M. K., El-Mahrouk, M. E., Taha, N., Bayoumi, Y., et al. (2022). An academic and technical overview on plant micropropagation challenges. Horticulturae 8 (8), 677. doi: 10.3390/horticulturae8080677
Ahmed, M. R., Anis, M., Alatar, A. A., Faisal, M. (2017). In vitro clonal propagation and evaluation of genetic fidelity using RAPD and ISSR marker in micropropagated plants of Cassia alata L.: a potential medicinal plant. Agrofor. Syst. 91, 637–647. doi: 10.1007/s10457-016-9992-1
Alsafran, M., Wickramanayake, K., Usman, K., Ahmed, T. (2022). Efficient shoot regeneration of medicinal plant Haplophyllum tuberculatum by direct and indirect organogenesis and genetic fidelity assessment using Inter Simple Sequence Repeats markers. Front. Plant Sci. 13. doi: 10.3389/fpls.2022.995825
Amiri, S., Panahi, B., Mohammadi, R., Fattahi, F. (2020). Effect of plant growth regulator combination on direct in vitro regeneration of persian lilac (Melia azedarach L.). Proc. Natl. Acad. Sci. India B - Biol. Sci. 90, 261–265. doi: 10.1007/s40011-019-01099-5
Atta, R., Laurens, L., Boucheron-Dubuisson, E., Guivarc’h, A., Carnero, E., Giraudat-Pautot, V., et al. (2009). Pluripotency of Arabidopsis xylem pericycle underlies shoot regeneration from root and hypocotyl explants grown in vitro. Plant J. 57, 626–644. doi: 10.1111/j.1365-313X.2008.03715.x
Backiyarani, S., Uma, S., Saranya, S., Durai, P., Eugin Perianayagaraj, S., Selvaraj, V., et al. (2021). Multiple shoot induction in zygotic embryos: a strategy for acceleration of banana breeding. Plant Cell Tissue Organ Cult 147, 339–350. doi: 10.1007/s11240-021-02127-x
Bednarek, P. T., Orłowska, R. (2020). Plant tissue culture environment as a switch-key of (epi)genetic changes. Plant Cell Tissue Organ Culture (PCTOC) 140, 245–257. doi: 10.1007/s11240-019-01724-1
Carlquist, S. (1996). Wood anatomy of akaniaceae and bretschneideraceae: A case of near-identity and its systematic implications. Systematic Bot. 21, 607–616. doi: 10.2307/2419618
Chen, H., Qiao, Q., Liu, H., Wen, X., Harris, A. J. (2020). “Reintroduction of Bretschneidera sinensis Hemsl., an Endangered Endemic Tree,” in Conservation and Reintroduction of Rare and Endangered Plants in China. Ed. Ren, H. (Springer Singapore, Singapore).
Cheng, Z. J., Wang, L., Sun, W., Zhang, Y., Zhou, C., Su, Y. H., et al. (2013). Pattern of auxin and cytokinin responses for shoot meristem induction results from the regulation of cytokinin biosynthesis by AUXIN RESPONSE FACTOR3. Plant Physiol. 161, 240–251. doi: 10.1104/pp.112.203166
Dai, X., Liu, Z., Qiao, M., Li, J., Li, S., Xiang, F. (2017). ARR12 promotes de novo shoot regeneration in Arabidopsis thaliana via activation of WUSCHEL expression. J. Integr. Plant Biol. 59, 747–758. doi: 10.1111/jipb.12567
Duarte-Aké, F., De-la-Peña, C. (2021). High cytokinin concentration and nutrient starvation trigger DNA methylation changes in somaclonal variants of Agave angustifolia Haw. Ind. Crops Products 172, 114046. doi: 10.1016/j.indcrop.2021.114046
Eeckhaut, T., Van Houtven, W., Bruznican, S., Leus, L., Van Huylenbroeck, J. (2020). Somaclonal variation in chrysanthemum × morifolium protoplast regenerants. Front. Plant Sci. 11. doi: 10.3389/fpls.2020.607171
El-Sayed, M., Aly, U. I., Mohamed, M. S., Rady, M. R. (2020). In vitro regeneration and molecular characterization of Jatropha curcas plant. Bull. Natl. Res. Cent 44, 70. doi: 10.1186/s42269-020-00320-0
Erişen, S., Kurt-Gür, G., Servi, H. (2020). In vitro propagation of Salvia sclarea L. by meta-Topolin, and assessment of genetic stability and secondary metabolite profiling of micropropagated plants. Ind. Crops Prod 157, 112892. doi: 10.1016/j.indcrop.2020.112892
Esposito-Polesi, N. P., de Oliveira, L. S., Baccarin, F. J. B., de Almeida, C. V., de Almeida, M. (2020). Different culture conditions applied to in vitro shoot multiplication of two Eucalyptus benthamii explant sources. J. For. Res. 31, 857–869. doi: 10.1007/s11676-018-0816-1
Fan, M., Xu, C., Xu, K., Hu, Y. (2012). LATERAL ORGAN BOUNDARIES DOMAIN transcription factors direct callus formation in Arabidopsis regeneration. Cell Res. 22, 1169–1180. doi: 10.1038/cr.2012.63
Fernandes, P., Tedesco, S., Vieira da Silva, I., Santos, C., MaChado, H., Lourenço Costa, R. (2020). A new clonal propagation protocol develops quality root systems in chestnut. Forests 11 (8), 826. doi: 10.3390/f11080826
Ferreira, M.d., Rocha, A., Nascimento, F., Oliveira, W.D.d., Soares, J.M.d., et al. (2023). The role of somaclonal variation in plant genetic improvement: A systematic review. Agronomy 13 (3), 730. doi: 10.3390/agronomy13030730
Fu, S., Hu, H.-k., Zhang, Q., Fang, Y., Shen, Z.-X., Gao, Y. (2012). In vitro propagation of katsura tree ( Cercidiphyllum japonicum Sieb. Et Zucc), an endangered plant in China. Afr J. Biotechnol. 11, 14911–14919. doi: 10.5897/AJB12.1957
Gawel, N. J., Jarret, R. L. (1991). A modified CTAB DNA extraction procedure for Musa and Ipomoea. Plant Mol. Bio. Rep. 9, 262–266. doi: 10.1007/BF02672076
Guo, Z., Long, Y., Xiao, G. (2007). Tissue culture and rapid propagation of bretschneidera sinensis hemsl. Plant Physiol. J. 239, 127.
Gupta, P. K., Durzan, D. J. (1985). Shoot multiplication from mature trees of Douglas-fir (Pseudotsuga menziesii) and sugar pine (Pinus lambertiana). Plant Cell Rep. 4, 177–179. doi: 10.1007/bf00269282
Hazubska-Przybył, T. (2019). Propagation of juniper species by plant tissue culture: A mini-review. Forests 10 (11), 1028. doi: 10.3390/f10111028
Ioannidis, K., Tomprou, I., Mitsis, V., Koropouli, P. (2022). Genetic evaluation of in vitro micropropagated and regenerated plants of cannabis sativa L. Using SSR molecular markers. Plants 11 (19), 2569. doi: 10.3390/plants11192569
Isah, T. (2015). Rethinking Ginkgo biloba L.: Medicinal uses and conservation. Pharmacogn Rev. 9, 140–148. doi: 10.4103/0973-7847.162137
Kadapatti, S. S., Murthy, H. N. (2021). Rapid plant regeneration, analysis of genetic fidelity, and neoandrographolide content of micropropagated plants of Andrographis alata (Vahl) Nees. J. Genet. Eng. Biotechnol. 19, 20. doi: 10.1186/s43141-021-00122-5
Kareem, A., Durgaprasad, K., Sugimoto, K., Du, Y., Pulianmackal, A. J., Trivedi, Z. B., et al. (2015). PLETHORA genes control regeneration by a two-step mechanism. Curr. Biol. 25, 1017–1030. doi: 10.1016/j.cub.2015.02.022
Kim, C., Dai, W. (2020). Plant regeneration of red raspberry (Rubus idaeus) cultivars ‘Joan J’ and ‘Polana’. In Vitro Cell. Dev. Biol. Plant 56, 390–397. doi: 10.1007/s11627-019-10051-1
Kim, J. Y., Yang, W., Forner, J., Lohmann, J. U., Noh, B., Noh, Y. S. (2018). Epigenetic reprogramming by histone acetyltransferase HAG1/AtGCN5 is required for pluripotency acquisition in Arabidopsis. EMBO J. 37, e98726. doi: 10.15252/embj.201798726
Li, Y. Y., Chen, X. Y., Zhang, X., Wu, T. Y., Lu, H. P., Cai, Y. W. (2005). Genetic differences between wild and artificial populations of Metasequoia glyptostroboides: implications for species recovery. Conserv. Biol. 19, 224–231. doi: 10.1111/j.1523-1739.2005.00025.x
Lin, W., Xiao, X., Zhang, H., Li, Y., Liu, S., Sun, W., et al. (2019). Whole-genome bisulfite sequencing reveals a role for DNA methylation in variants from callus culture of pineapple (Ananas comosus L.). Genes 10 (11), 877. doi: 10.3390/genes10110877
Liu, C. M., Li, B., Shen, Y. H., Zhang, W. D. (2010). Heterocyclic compounds and aromatic diglycosides from Bretschneidera sinensis. J. Nat. Prod 73, 1582–1585. doi: 10.1021/np1002934
Liu, F., Xu, G., Li, T., Luo, C. (2021). Characterization and development of SSR markers from transcriptome sequence of Bretschneidera sinensis. Hemsl. Plant Sci. J. 39, 288–296. doi: 10.11913/PSJ.2095-0837.2021.30288
Liu, H.-L., Harris, A. J., Wang, Z.-F., Chen, H.-F., Li, Z.-A., Wei, X. (2022). The genome of the Paleogene relic tree Bretschneidera sinensis: insights into trade-offs in gene family evolution, demographic history, and adaptive SNPs. DNA Res. 29 (1), dsac003. doi: 10.1093/dnares/dsac003
Liu, J., Hu, X., Qin, P., Prasad, K., Hu, Y., Xu, L. (2018). The WOX11-LBD16 pathway promotes pluripotency acquisition in callus cells during de novo shoot regeneration in tissue culture. Plant Cell Physiol. 59, 734–743. doi: 10.1093/pcp/pcy010
Lloyd Gregory, M. B. (1980). Commercially-feasible micropropagation of mountain laurel, Kalmia latifolia, by use of shoot-tip culture. Plant Prop Soc. Proc. 30, 421–427.
Luo, Y., Zheng, K., Liu, X., Tao, J., Sun, X., Deng, Y., et al. (2023). In vitro propagation and genetic uniformity assessment of Manglietiastrum sinicum: A critically endangered magnoliaceae species. Plants 12, 2500. doi: 10.3390/plants12132500
Ma, C., Goddard, A., Peremyslova, E., Duan, C., Jiang, Y., Nagle, M., et al. (2022). Factors affecting in vitro regeneration in the model tree Populus trichocarpa I. Medium, environment, and hormone controls on organogenesis. In Vitro Cell. Dev. Biol. - Plant 58, 837–852. doi: 10.1007/s11627-022-10301-9
Mehta, R., Sharma, V., Sood, A., Sharma, M., Sharma, R. K. (2011). Induction of somatic embryogenesis and analysis of genetic fidelity of in vitro-derived plantlets of Bambusa nutans Wall., using AFLP markers. Eur. J. For Res. 130, 729–736. doi: 10.1007/s10342-010-0462-4
Meng, W. J., Cheng, Z. J., Sang, Y. L., Zhang, M. M., Rong, X. F., Wang, Z. W., et al. (2017). Type-B ARABIDOPSIS RESPONSE REGULATORs specify the shoot stem cell niche by dual regulation of WUSCHEL. Plant Cell 29, 1357–1372. doi: 10.1105/tpc.16.00640
Montaut, S., Zhang, W. D., Nuzillard, J.-M., De Nicola, G. R., Rollin, P. (2015). Glucosinolate diversity in bretschneidera sinensis of chinese origin. J. Natural Products 78, 2001–2006. doi: 10.1021/acs.jnatprod.5b00338
Murashige, T., Skoog, F. K. (1962). A revised medium for the growth and bioassay with tobacco tissue cultures. Physiol. Plant 15, 473–497. doi: 10.1111/j.1399-3054.1962.tb08052.x
Nowakowska, K., Pińkowska, A., Siedlecka, E., Pacholczak, A. (2022). The effect of cytokinins on shoot proliferation, biochemical changes and genetic stability of Rhododendron ‘Kazimierz Odnowiciel’ in the in vitro cultures. Plant Cell Tissue Organ Cult 149, 675–684. doi: 10.1007/s11240-021-02206-z
Osorio, C. R., Velásquez, F., Correal, A. G., Bonilla, J. M. T., Trujillo, A. I. U. (2018). In vitro propagation of avocado (Persea americana Mill. cv. Hass) through morphogenesis. Acta Agron. 67, 160–167. doi: 10.15446/acag.v67n1.61474
Phillips, G. C., Garda, M. (2019). Plant tissue culture media and practices: an overview. In Vitro Cell. Dev. Biol. Plant 55, 242–257. doi: 10.1007/s11627-019-09983-5
Pillai, P. P., Sajan, J. S., Menon, K. M., Panicker Jayakumar, K. S., Subramoniam, A. (2012). ISSR analysis reveals high intraspecific variation in Rauvolfia serpentina L. – A high value medicinal plant. Biochem. Syst. Ecol. 40, 192–197. doi: 10.1016/j.bse.2011.10.019
Qi, Q., Hong-Feng, C., Fu-Wu, X., Dong-Ming, L., Xiao-Gai, H. (2009). Seed germination protocol for the threatened plant species, Bretschneidera sinensis Hemsl. Seed Sci. Technol. 37, 70–78. doi: 10.15258/sst.2009.37.1.09
Qiao, Q., Chen, H., Xing, F. W., Wang, F., Zhong, W.-Y., Wen, X., et al. (2012). Pollination ecology of Bretschneidera sinensis (hemsley), a rare and endangered tree in China. Pak. J. Bot. 44, 1897–1903.
Romero, E. J., Hickey, L. J. (1976). A fossil leaf of akaniaceae from paleocene beds in Argentina. Bull. Torrey Bot. Club 103, 126–131. doi: 10.2307/2484888
Savitikadi, P., Jogam, P., Rohela, G. K., Ellendula, R., Sandhya, D., Allini, V. R., et al. (2020). Direct regeneration and genetic fidelity analysis of regenerated plants of Andrographis echioides (L.) - An important medicinal plant. Ind. Crops Products 155, 112766. doi: 10.1016/j.indcrop.2020.112766
Shang, C., Li, E., Yu, Z., Lian, M., Chen, Z., Liu, K., et al. (2022). Chloroplast genomic resources and genetic divergence of endangered species Bretschneidera sinensis (Bretschneideraceae). Front. Ecol. Evol. 10. doi: 10.3389/fevo.2022.873100
Shekhawat, M. S., Manokari, M. (2016). In vitro propagation, micromorphological studies and ex vitro rooting of cannon ball tree (Couroupita guianensis aubl.): a multipurpose threatened species. Physiol. Mol. Biol. Plants 22, 131–142. doi: 10.1007/s12298-015-0335-x
Shekhawat, M. S., Mehta, S. R., Manokari, M., Priyadharshini, S., Badhepuri, M. K., Jogam, P., et al. (2021). Morpho-anatomical and physiological changes of Indian sandalwood (Santalum album L.) plantlets in ex vitro conditions to support successful acclimatization for plant mass production. Plant Cell Tissue Organ Cult 147, 423–435. doi: 10.1007/s11240-021-02136-w
Song, W., Song, Y., Liu, X., Zhang, X., Xin, R., Duan, S., et al. (2023). Improvement of culture conditions and plant growth regulators for in vitro callus induction and plant regeneration in paeonia lactiflora pall. Plants 12 (23), 3968. doi: 10.3390/plants12233968
Srinivasan, P., Raja, H. D., Tamilvanan, R. (2021). Efficient in vitro plant regeneration from leaf-derived callus and genetic fidelity assessment of an endemic medicinal plant Ranunculus wallichianus Wight & Arnn by using RAPD and ISSR markers. Plant Cell Tissue Organ Cult 147, 413–420. doi: 10.1007/s11240-021-02134-y
Stroud, H., Ding, B., Simon, S. A., Feng, S., Bellizzi, M., Pellegrini, M., et al. (2013). Plants regenerated from tissue culture contain stable epigenome changes in rice. eLife 2, e00354. doi: 10.7554/eLife.00354
Su, Y. H., Zhou, C., Li, Y. J., Yu, Y., Tang, L. P., Zhang, W. J., et al. (2020). Integration of pluripotency pathways regulates stem cell maintenance in the Arabidopsis shoot meristem. Proc. Natl. Acad. Sci. U.S.A. 117, 22561–22571. doi: 10.1073/pnas.2015248117
Sugimoto, K., Jiao, Y., Meyerowitz, E. M. (2010). Arabidopsis regeneration from multiple tissues occurs via a root development pathway. Dev. Cell 18, 463–471. doi: 10.1016/j.devcel.2010.02.004
Sultana, K. W., Das, S., Chandra, I., Roy, A. (2022). Efficient micropropagation of Thunbergia coccinea Wall. and genetic homogeneity assessment through RAPD and ISSR markers. Sci. Rep. 12, 1683. doi: 10.1038/s41598-022-05787-7
Thorpe, T. A., Harry, I. S. (1990). “Special Problems and Prospects in the Propagation of Woody Species,” in Plant Aging: Basic and Applied Approaches. Eds. Rodríguez, R., Tamés, R. S., Durzan, D. J. (Springer US, Boston, MA).
Tommasi, F., Scaramuzzi, F. (2004). In vitro Propagation of Ginkgo biloba by Using Various Bud Cultures. Biol. Plant 48, 297–300. doi: 10.1023/B:BIOP.0000033460.75432.d1
Tu, Q., Wu, T., Zhao, L., Liu, Y., Liu, R., Zhang, Z. (2012). Features of leaf appendages of Bretschneidera sinensis in different development stages. Plant Divers. 34, 248–256. doi: 10.3724/SP.J.1143.2012.11184
Varapparambath, V., Mathew, M. M., Shanmukhan, A. P., Radhakrishnan, D., Kareem, A., Verma, S., et al. (2022). Mechanical conflict caused by a cell-wall-loosening enzyme activates de novo shoot regeneration. Dev. Cell 57, 2063–2080.e10. doi: 10.1016/j.devcel.2022.07.017
Wang, J., Tian, C., Zhang, C., Shi, B., Cao, X., Zhang, T. Q., et al. (2017). Cytokinin signaling activates WUSCHEL expression during axillary meristem initiation. Plant Cell 29, 1373–1387. doi: 10.1105/tpc.16.00579
Wang, M. N., Duan, L., Qiao, Q., Wang, Z. F., Zimmer, E. A., Li, Z. C., et al. (2018). Phylogeography and conservation genetics of the rare and relict Bretschneidera sinensis (Akaniaceae). PloS One 13, e0189034. doi: 10.1371/journal.pone.0189034
Wang, Y., He, X., Yu, F. (2022). Non-host plants: Are they mycorrhizal networks players? Plant Diversity 44, 127–134. doi: 10.1016/j.pld.2021.06.005
Wei, F., Zhao, F. F., Tian, B. M. (2017). In vitro regeneration of Populus tomentosa from petioles. J. For. Res. 28, 465–471. doi: 10.1007/s11676-016-0319-x
Wu, L. Y., Shang, G. D., Wang, F. X., Gao, J., Wan, M. C., Xu, Z. G., et al. (2022). Dynamic chromatin state profiling reveals regulatory roles of auxin and cytokinin in shoot regeneration. Dev. Cell 57, 526–542.e7. doi: 10.1016/j.devcel.2021.12.019
Xiong, Y., Chen, S., Guo, B., Niu, M., Zhang, X., Li, Y., et al. (2020). An efficient micropropagation protocol for Metasequoia glyptostroboides Hu et Cheng from shoot segments of 2-year-old trees. Trees 34, 307–313. doi: 10.1007/s00468-019-01905-7
Xu, C., Cao, H., Zhang, Q., Wang, H., Xin, W., Xu, E., et al. (2018). Control of auxin-induced callus formation by bZIP59-LBD complex in Arabidopsis regeneration. Nat. Plants 4, 108–115. doi: 10.1038/s41477-017-0095-4
Yan, X., Wang, K., Zheng, K., Zhang, L., Ye, Y., Qi, L., et al. (2023). Efficient organogenesis and taxifolin production system from mature zygotic embryos and needles in larch. Forestry Res. 3, 1. doi: 10.48130/FR-2023-0004
Yang, J., Cai, L., Liu, D., Chen, G., Gratzfeld, J., Sun, W. (2020). China's conservation program on Plant Species with Extremely Small Populations (PSESP): Progress and perspectives. Biol. Conserv. 244, 108535. doi: 10.1016/j.biocon.2020.108535
Yuan, L., Ma, S., Liu, K., Wang, T., Xiao, D., Zhang, A., et al. (2023). High frequency adventitious shoot regeneration from hypocotyl-derived callus of Glyptostrobus pensilis, a critically endangered plant. Plant Cell Tissue Organ Cult 152, 139–149. doi: 10.1007/s11240-022-02396-0
Zdravković-Korać, S., Milojević, J., Belić, M., Ćalić, D. (2022). Tissue culture response of ornamental and medicinal aesculus species—A review. Plants 11 (3), 277. doi: 10.3390/plants11030277
Zhai, N., Pan, X., Zeng, M., Xu, L. (2023). Developmental trajectory of pluripotent stem cell establishment in Arabidopsis callus guided by a quiescent center-related gene network. Development 150 (5), dev200879. doi: 10.1242/dev.200879
Zhai, N., Xu, L. (2021). Pluripotency acquisition in the middle cell layer of callus is required for organ regeneration. Nat. Plants 7, 1453–1460. doi: 10.1038/s41477-021-01015-8
Zhang, H., Du, X., Dong, C., Zheng, Z., Mu, W., Zhu, M., et al. (2022). Genomes and demographic histories of the endangered Bretschneidera sinensis (Akaniaceae). GigaScience 11, giac050. doi: 10.1093/gigascience/giac050
Keywords: Bretschneidera sinensis, mature zygotic embryo, organogenesis, genetic fidelity, molecular markers
Citation: Yan X, Zheng K, Li P, Zhong X, Zhu Z, Zhou H and Zhu M (2024) An efficient in vitro organogenesis protocol for the endangered relic tree species Bretschneidera sinensis and genetic fidelity assessment using DNA markers. Front. Plant Sci. 15:1259925. doi: 10.3389/fpls.2024.1259925
Received: 17 July 2023; Accepted: 14 March 2024;
Published: 10 April 2024.
Edited by:
Chenghao Li, Northeast Forestry University, ChinaReviewed by:
Xiaomei Deng, South China Agricultural University, ChinaZhuo Renying, Chinese Academy of Forestry, China
Copyright © 2024 Yan, Zheng, Li, Zhong, Zhu, Zhou and Zhu. This is an open-access article distributed under the terms of the Creative Commons Attribution License (CC BY). The use, distribution or reproduction in other forums is permitted, provided the original author(s) and the copyright owner(s) are credited and that the original publication in this journal is cited, in accordance with accepted academic practice. No use, distribution or reproduction is permitted which does not comply with these terms.
*Correspondence: Mulan Zhu, mlzhu@cemps.ac.cn