When the woolly rhinoceroses roamed East Asia: a review of isotopic paleoecology of the genus Coelodonta from the Tibetan Plateau to northern Eurasia
- 1Key Laboratory of Vertebrate Evolution and Human Origins of Chinese Academy of Sciences, Institute of Vertebrate Palaeontology and Palaeoanthropology, Chinese Academy of Sciences, Beijing, China
- 2State Key Laboratory of Palaeobiology and Stratigraphy, Nanjing Institute of Geology and Palaeontology, Chinese Academy of Sciences, Nanjing, China
- 3University of Chinese Academy of Sciences, Beijing, China
East Asia, being the evolutionary center of Coelodonta, offers a unique opportunity to explore the spatiotemporal paleoecologies of this genus. This study utilized bulk and serial stable carbon and oxygen isotope analyses on the Coelodonta nihowanensis and other ungulates from the Longdan fauna as well as two modern goats in the Linxia Basin, aiming to explore the paleoecology of C. nihowanensis at the periphery of the Tibetan Plateau during the Early Pleistocene. The isotopic results of these mammals indicated that C3 vegetation was possibly a major component of the local environment in the Linxia Basin, which was influenced by a seasonal summer monsoon. C. nihowanensis specimens in the Longdan fauna were possibly mixed feeders, as indicated by the wide distribution of their δ13C values and the intermediate δ18O values compared to other coexisting ungulates. Moreover, the comparison of the δ13C and δ18O values of similar taxa from the Linxia and Nihewan basins has revealed spatiotemporal differences in the paleoclimate and paleoenvironments of these two regions throughout the Early Pleistocene. This provides a holistic framework for understanding the paleoecology of the C. nihowanensis. The isotopic results of the C. nihowanensis in the Linxia and Nihewan basins suggested varied foraging ecologies across different sites and time spans during the Early Pleistocene. This underscores the adaptability of the C. nihowanensis to diverse environments from west to east in northern China. By scrutinizing the evolutionary ecological history of the woolly rhinoceroses from its ancestor, Coelodonta thibetana, to its final form, Coelodonta antiquitatis, this study sheds light on the ecological adaptation of this genus from the Pliocene to Late Pleistocene, spanning its migration from the Tibetan Plateau to northern Eurasia.
1 Introduction
As the final representative of the woolly rhinoceroses, Coelodonta antiquitatis stands as an iconic species thriving on the mammoth steppe during the Late Pleistocene, with its distribution extending widely across northern Eurasia (Kahlke and Lacombat, 2008; Kahlke, 2014). However, East Asia has long been considered the cradle and evolutional center of the genus Coelodonta.
Deng et al. (2011) documented the earliest known ancestor of the woolly rhinoceroses, Coelodonta thibetana, originating from the Zanda Basin in the southwestern Tibetan Plateau at 5.08 Ma (Deng et al., 2011; Wang X. et al., 2013). This species then evolved into Coelodonta nihowanensis, which mainly thrived in northern China during the Early Pleistocene (Deng, 2002, 2008; Jin et al., 2021). C. nihowanensis in the Linxia Basin was considered to be the earliest woolly rhinoceros (2.5–2.2 Ma) prior to the discovery of the C. thibetana (Qiu et al., 2004; Deng, 2006; Zan et al., 2016; Deng et al., 2023). C. nihowanensis then dispersed out the Tibetan region, and it was commonly found in multiple sites in northern China, including the Nihewan Basin and Jinyuan Cave during the Early Pleistocene (e.g. Tong and Wang, 2014; Chen et al., 2017; Dong et al., 2021; Chen et al., 2023). C. nihowanensis gradually evolved into Coelodonta tologoijensis and was discovered in Russia and Mongolia during the early Middle Pleistocene. Its occurrence in Europe is still in debate (Kahlke and Lacombat, 2008; Uzunidis et al., 2022). Coelodonta then terminated in C. antiquitatis and its distribution extended over approximately 190° of longitude and 40° of latitude (Kahlke, 1999; Stefaniak et al., 2023). Through the evolutionary history of the woolly rhinoceroses, the genus Coelodonta was restricted in East Asia for more than 4 million years. Therefore, delving into the paleoecological shifts within Coelodonta in East Asia becomes essential for a deeper comprehension of the interplay between its evolutionary dynamics and the paleoenvironmental changes.
The series of morphological traits of Coelodonta, including special nasal horn, a low position of the head, and complex tooth morphology, were all considered indicators of grazing in cold and dry environment (Fortelius, 1983; Kahlke and Lacombat, 2008; Deng et al., 2011). As the most emblematic member of Coelodonta, the paleoecology of C. antiquitatis was intensively explored through various methods, and it was evidenced as a cold-adapted grazer (e.g. Rivals et al., 2010; Pushkina et al., 2020). The stable isotope analysis was applied on C. thibetana and C. nihowanensis in the Zanda and Nihewan basins. C. thibetana in the Zanda Basin fed on C3 vegetation in an environment dominated by C3 plants (Wang Y. et al., 2013), and C. nihowanensis in the Nihewan Basin displayed different foraging ecologies at different sites (Xu et al., 2021; Xu et al., 2023). The presence of C. nihowanensis in the Linxia Basin suggests a connection between the mid-Pliocene Zanda Basin and the Early Pleistocene Nihewan Basin (Deng et al., 2023). This provides an opportunity to explore the paleoecological changes of Coelodonta as they migrated out of the Tibetan Plateau. The previous study explored the paleoecology of Cenozoic rhinoceroses in the Linxia Basin; however, only one C. nihowanensis specimen was analyzed and identified as a grazer in the Longdan fauna (Biasatti et al., 2018). It is evident that more specimens are required to investigate the dietary ecology of C. nihowanensis in the Linxia Basin. Furthermore, all isotopic data from East Asia should be discussed from the perspective of the Coelodonta evolution.
In this study, we performed stable carbon and oxygen isotope analyses on C. nihowanensis and other ungulates from the Longdan fauna to investigate the paleoecology of C. nihowanensis in the Linxia Basin during the Early Pleistocene. Additionally, we conducted an extensive review of all published isotopic data pertaining to Coelodonta and coexisting mammals, aiming to provide a deeper understanding of the paleoecological shifts experienced by Coelodonta in East Asia.
2 Carbon and oxygen isotopes in tooth enamel
Higher plants are generally divided into C3 (including virtually all trees, most shrubs and herbs, and cool-season grasses), C4 (warm season grasses), and CAM (succulents) plants according to different photosynthesis pathways (Troughton and Card, 1975; O’Leary, 1981; Farquhar et al., 1989). Modern C3 plants have an average δ13C value of −25‰, while C4 plants have an average δ13C value of −13‰ (Bender, 1968; Smith and Epsten, 1971; Kohn and Cerling, 2002). Additionally, water use efficiency, the canopy effect, natural physiology, and abiotic factors can impact the δ13C values of plant tissues (van der Merwe and Medina, 1991; Quade et al., 1995; Peuke et al., 2006; Kohn, 2010). When plants are directly consumed by mammals, isotopic fractionation occurs during the process of tissue formation. The enrichment factors between plant consumption and tooth enamel bioapatite are roughly 14‰ for herbivores, although they may be slightly influenced by variations in their body sizes and digestive strategies (Ambrose and Norr, 1993; Cerling and Harris, 1999; Kohn and Cerling, 2002; Tejada-Lara et al., 2018; Cerling et al., 2021). A tooth enamel with a δ13C value of less than −9‰ generally indicates a pure C3 diet, while a δ13C value greater than −2‰ would demonstrate a pure C4 diet (Cerling et al., 1997). Nevertheless, in water-stressed environments, a conservative enamel-δ13C value cut-off for a pure C3 diet in fossil herbivores should likely be −7‰ or even −6.5‰ (Cerling et al., 1997; Wang et al., 2008a). Therefore, the δ13C values of mammals are valuable for reconstructing their paleodiet and paleoecology. Nonetheless, recent researches have uncovered that the relationships between herbivore assemblages and the vegetation landscapes are complicated (Negash and Barr, 2023; Sokolowski et al., 2023), underscoring the importance to avoid simplistic interpretations of paleolandscapes based solely on the δ13C values of fossil herbivores.
The δ18O values in the bioapatite of the mammal bones and teeth are primarily determined by the source of water consumed and their foraging ecology (Bryant and Froelich, 1995; Pederzani and Britton, 2019). For large herbivores relying heavily on drinking water, the δ18O values derived from tooth enamel directly reflect the δ18O values they consume, which can vary due to factors, such as local temperature, precipitation, and humidity (Dutton et al., 2005; Pederzani and Britton, 2019). In general, tree leaves exhibit higher δ18O values than grasses due to increased transpiration of water (Koch et al., 1989; Quade et al., 1995). Consequently, herbivores relying on such foliage typically demonstrate higher δ18O values than those consuming grasses.
Mammalian teeth form incrementally from the top to the bottom of the tooth crown during the period of tooth growth (Hillson, 2005). Once tooth enamel is fully mineralized, it remains unaltered throughout the lifespan of mammals, thereby preserving the isotopic sequence formed during its development (Suga, 1979). Consequently, bulk sampling of the entire height of tooth enamel can be used to reconstruct the average diet and ecology during the tooth formation period, while serial sampling of the tooth enamel along the direction of enamel growth can offer detailed insights into the dietary and ecological changes of mammals (Kohn et al., 1998; Balasse, 2002; Blumenthal et al., 2014, Blumenthal et al., 2019; Li et al., 2020). However, enamel mineralization progresses in a non-linear manner, following distinctive trajectories within the enamel layers that do not consistently align with incremental growth lines (Suga, 1979; Smith et al., 2003). Therefore, conventional serial sampling method often yields composite samples from multiple enamel layers, which may potentially dampen seasonal signals (Sharp and Cerling, 1998; Higgins and MacFadden, 2004; Green et al., 2018).
3 Materials and methods
3.1 Geological settings and sample selection
The Linxia Basin, located on the northeastern edge of the Tibetan Plateau, is well known for its thick and continuous Late Cenozoic deposits and its exceptionally rich and diverse mammalian fossils (Deng et al., 2013). The Longdan, the first Early Pleistocene site in China discovered from loess, belongs to the Wucheng Formation, characterized by yellowish hard loess with layers of calcite concretions (Qiu et al., 2002; Qiu et al., 2004). Magnetostratigraphic dating had determined the age of the Longdan fauna to be 2.5–2.2 Ma (Zan et al., 2016). This fauna has yielded abundant fossils of 33 mammalian species, including Equus eisenmannae, Proboscidipparion sinense, C. nihowanensis, Leptobos brevicornis, and a diverse range of carnivores (Qiu et al., 2004). The mammalian fossils in the Longdan fauna exhibit slightly more primitive features than those of the Early Pleistocene Nihewan fauna (Qiu et al., 2004). The Nihewan fauna represents another significant Early Pleistocene mammalian assemblage in northern China, preserving abundant C. nihowanensis fossils, and its geological age are 2.4–1.8 Ma or 2.2–1.7 Ma (Qiu, 2000; Liu et al., 2012; Farjand et al., 2023a; Farjand et al., 2023b). E. eisenmannae in the Longdan fauna was one of the earliest and most primitive Equus in Eurasia, while C. nihowanensis in this fauna was initially regarded as the most primitive woolly rhinoceros before the discovery of the C. thibetana (Qiu et al., 2004; Deng, 2006).
3.2 Materials
27 fossil tooth samples were collected from the Longdan fauna, including the woolly rhinoceros C. nihowanensis (n=7), the deer Nipponicervus longdanensis (n=4), Bovinae (L. brevicornis and Hemibos gracilis) (n=7), and the gazelle Gazella cf. blacki (n=9). These specimens were housed in the Institute of Vertebrate Paleontology and Paleoanthropology and the Hezheng Paleozoological Museum in China. However, the precise stratigraphic levels of these tooth specimens were not recorded. Two modern goats were collected as modern references. Among them, 8 fossil samples and 2 modern goats were serially sampled. In total, 103 specimens were collected.
3.3 Stable isotope analysis
First, the teeth were cleaned by removing a very thin (~0.1 mm) layer of outer enamel using a diamond-tipped dental burr. For bulk samples, enamel powder was collected from the entire range of tooth heights to obtain an average value. Well-preserved teeth with complete tooth crown height were chosen for serial sampling. Each serial sample was obtained by drilling with a 1–2 mm-wide groove mostly perpendicular to the tooth growth axis, penetrating through the enamel layer’s thickness at intervals of approximately 1–2 mm. These sampling methods were designed to encompass the full crown height of each tooth piece. Approximately 12–15 mg of enamel powder was drilled and collected in a 1.5-ml centrifuge tube for each sample.
Second, all the enamel powder underwent pretreatment with 2.5% NaOCl followed by 1 mol/L acetic acid. Subsequently, 3–5 mg of powdered enamel was analyzed at the Laboratory for Stable Isotope Geochemistry, Institute of Geology and Geophysics, Chinese Academy of Sciences using a MAT253 IRMS coupled with a Gasbench unit. The international isotope standards of IAEA CO-8 and NBS-19 were interspersed with every run to establish the standard curve for isotopic calibration. Additionally, the in-house standard GBW04405 (limestone) and sample replicates were analyzed to monitor measurement uncertainty. Carbon and oxygen isotope values were expressed as δ13C and δ18O values relative to VPDB. The analytical precision, based on long-term repeated measurements of the standards and sample replicates, was less than ± 0.15‰ for δ13C values and ± 0.20‰ for δ18O values.
Assessment of fossil tooth preservation in the Longdan fauna, as established in previous studies (Biasatti et al., 2010; Ma et al., 2023), suggests that the specimens in this study from the same fauna and sediments are likely well preserved.
3.4 Data analyses
We utilized the carbon isotope enrichment factors proposed in the most recent study based on modern animal experiments (Cerling et al., 2021): 13.5‰ for non-coprophagous hindgut fermenter (such as equids, woolly rhinoceroses, and mammoths) and 14.5‰ for ruminant foregut fermenter (including cervids, gazelles, and bovids). For the collagen samples from the Late Pleistocene, we applied 5‰ as the isotope enrichment factor (Cerling and Harris, 1999).
The isotopic data collected from different sites in this study cover a range of regions, necessitating the consideration of factors such as latitude and altitude. Previous studies have identified a positive relationship between elevation and the δ13C values of C3 plants, possibly influenced by air pressure and temperature (Körner et al., 1991; Männel et al., 2007). Conversely, the latitudinal gradient for the δ13C values remains less definitive (Krajcarz et al., 2016). The positive correlation between elevation and the δ13C values of C3 plants has been noted in the modern Tibetan region as well (Wang et al., 2008b), which is pertinent to our studied locations. However, the relationship between δ13C values of C4 plants and the elevation gradients is unclear and may exhibit a negative correlation in the modern Tibetan region (Wang et al., 2008b). Additionally, no clear relationship was observed between δ18O values of precipitation and altitude in this region (Wang et al., 2008b).
Therefore, the relationship between δ13C values of C3 plants and altitude appears to be an important factor in this study when analyzing isotopic values across sites at different elevations. We adopted the altitude gradient as per Männel et al. (2007): δ13C-adj-alt= δ13C -(0.0011×altitude), where altitude in meters. By applying this correction, we mitigate the impact of altitude, normalizing all data to sea level (0 meter above sea level, asl) for comparability. However, due to the unclear association between δ13C values of C4 plants and elevation, we chose not to adjust data from the regions where C4 plant components were notably present.
Mann-Whitney U tests were conducted to assess statistical variations in isotopic values across distinct groups. These analyses were conducted in PAST 4.03 (Hammer et al., 2001). Data visualizations were created in EXCEL and RStudio (RStudio Team, 2016) using ggplot2 (Wickham, 2011) and ggpubr (Kassambara, 2020).
4 Results and interpretations
4.1 Bulk carbon and oxygen isotope analyses
The δ13C and δ18O values for each tooth sample (bulk samples and the mean values of each serial sample) are summarized in Table 1 and displayed in Figure 1 (see original data in Supplementary Table S1). In order to capture a complete paleoecological scenario of the Longdan fauna, our previous data of the E. eisenmannae and P. sinense were also included (Ma et al., 2023). Overall, the δ13C values of all the specimens in this study ranged from −13.3‰ to −7.9‰, and their δ18O values ranged between −12.5‰ to −4.6‰. In Figure 1, δ13C and δ18O values of the Longdan equid samples were also fall into these ranges (Ma et al., 2023). This indicated that C3 vegetation constituted a significant portion of the ungulates’ diet and played a substantial role in the local environment during the Early Pleistocene in the Linxia Basin.
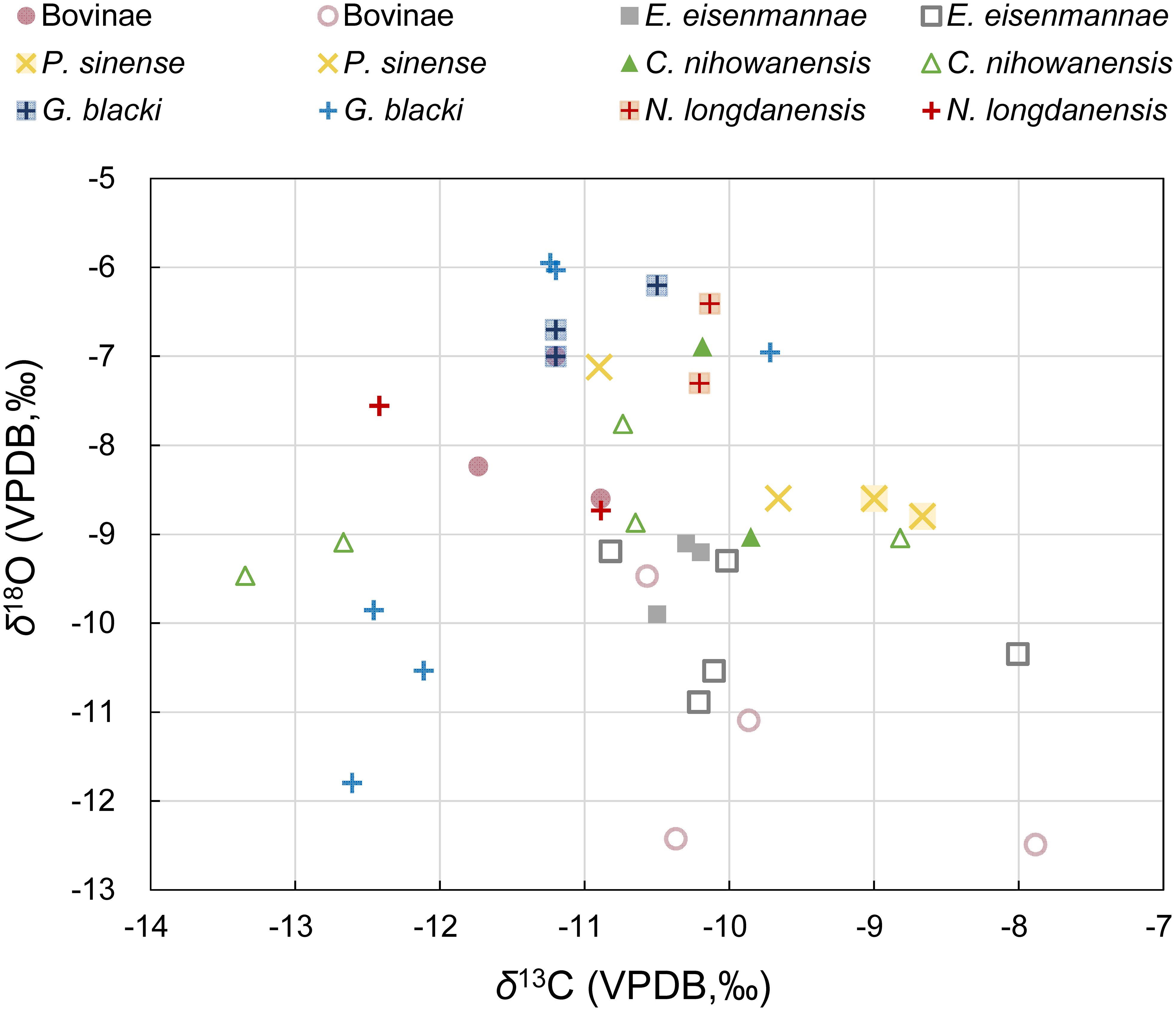
Figure 1 Stable isotopic data of the bulk samples (blank symbols) and the mean value of each serial sample (solid symbols) of the studied mammals (including data of P. sinense and E. eisenmannae from Ma et al., 2023).
The δ13C values of the four ungulates in this study were slightly lower than those of two equid species, which indicated that equids occupied more open environments than other mammals (Table 1; Figure 1). The results of the Mann-Whitney U tests revealed that the δ13C values of G. blacki were lower than P. sinense and E. eisenmannae (p-values = 0.015, 0.009), suggesting a possible preference for close environments in G. blacki compared to equids. Overall, four ungulates analyzed in this study exhibited subtle differences in δ13C values (Supplementary Table S2), indicating potential overlap in their food resources and habitats. The δ13C standard deviation was higher for C. nihowanensis specimens in comparison to other mammals (Table 1), indicating the diverse habitats they inhabited. In a previous study, one C. nihowanensis specimen with a δ13C value of −9.9‰ was classified as a grazer (Biasatti et al., 2018), within the range of δ13C values observed in our C. nihowanensis specimens (from −13.3‰ to −8.8‰, n=7).
As shown in Table 1, the mean δ18O values of the G. blacki and N. longdanensis specimens were higher than those of Bovinae and E. eisenmannae specimens, whereas the specimens of C. nihowanensis and P. sinense exhibited intermediate δ18O values. The results from the Mann-Whitney U tests revealed that the δ18O values of E. eisenmannae were lower than those of N. longdanensis and C. nihowanensis (refer to Supplementary Table S3), with no discernible differences were observed in other groups. This suggested that E. eisenmannae specimens likely rely more heavily on meteoric water resources (Sponheimer and Lee-Thorp, 1999), while N. longdanensis and C. nihowanensis may access different water resources, potentially obtaining a portion of their water from tree leaves. In general, tree leaves exhibit higher δ18O values than grasses because trees experience greater transpiration of water through the leaves (Koch et al., 1989; Quade et al., 1995). Meanwhile, high standard deviations in δ18O values were observed in the specimens of Bovinae, G. blacki, and P. sinense, suggesting potential access to a diverse range of water resources (Table 1; Figure 1). Nevertheless, these varied δ18O values may be caused by the potential differences in the chronology of these specimens.
A discrepancy was noted in the isotopic results between the averaged serial samples and the bulk samples from the specimens of N. longdanensis, P. sinense, and Bovinae. This difference could be linked to potential sampling bias inherent in the two distinct sampling methods. Despite the bulk samples being designed to encompass the entire height of the tooth, challenges arise when dealing with fragmented or heavily worn teeth. Consequently, the stable isotopic values obtained from partial tooth heights could introduce bias and fail to accurately reflect the averaged isotopic values. Nevertheless, considering the subtle intra-tooth isotopic ranges in these serial samples, we propose that the data disparity probably reflects their varied isotopic ecologies. Furthermore, the potential differences in chronology could be another contributing factor to this data discrepancy, given the absence of precise stratigraphic levels for these tooth specimens.
4.2 Serial isotopic analysis
The serial isotopic patterns observed in these mammals indicated notable signals of the ecological and environmental seasonality. All eight fossil teeth examined in this study exhibited stable δ13C values, with intra-tooth δ13C values ranging from 0.8‰ to 1.6‰ (Supplementary Table S1). This possibly indicated limited seasonal variations in the local vegetation. By comparison, the serial δ18O profiles of these ungulates displayed interspecific differences (Figure 2). The intra-tooth δ18O profiles of the large Bovinae (Supplementary Table S1; Figure 2) and equids (Biasatti et al., 2010; Ma et al., 2023) specimens demonstrated stable and monotonous patterns. The seasonal δ18O values observed in these large obligate drinkers may have been dampened due to their frequent consumption of water from large water bodies, which mitigated seasonal isotopic variations in local rainfall, owning to prolonged water residence times (Roberts et al., 2018; Pederzani and Britton, 2019). Alternatively, the stability and monotony observed in the intra-tooth δ18O profiles of the equid and bovid specimens might suggest migratory behavior. Consequently, their δ18O values may reflect a combination of water sources from various locations.
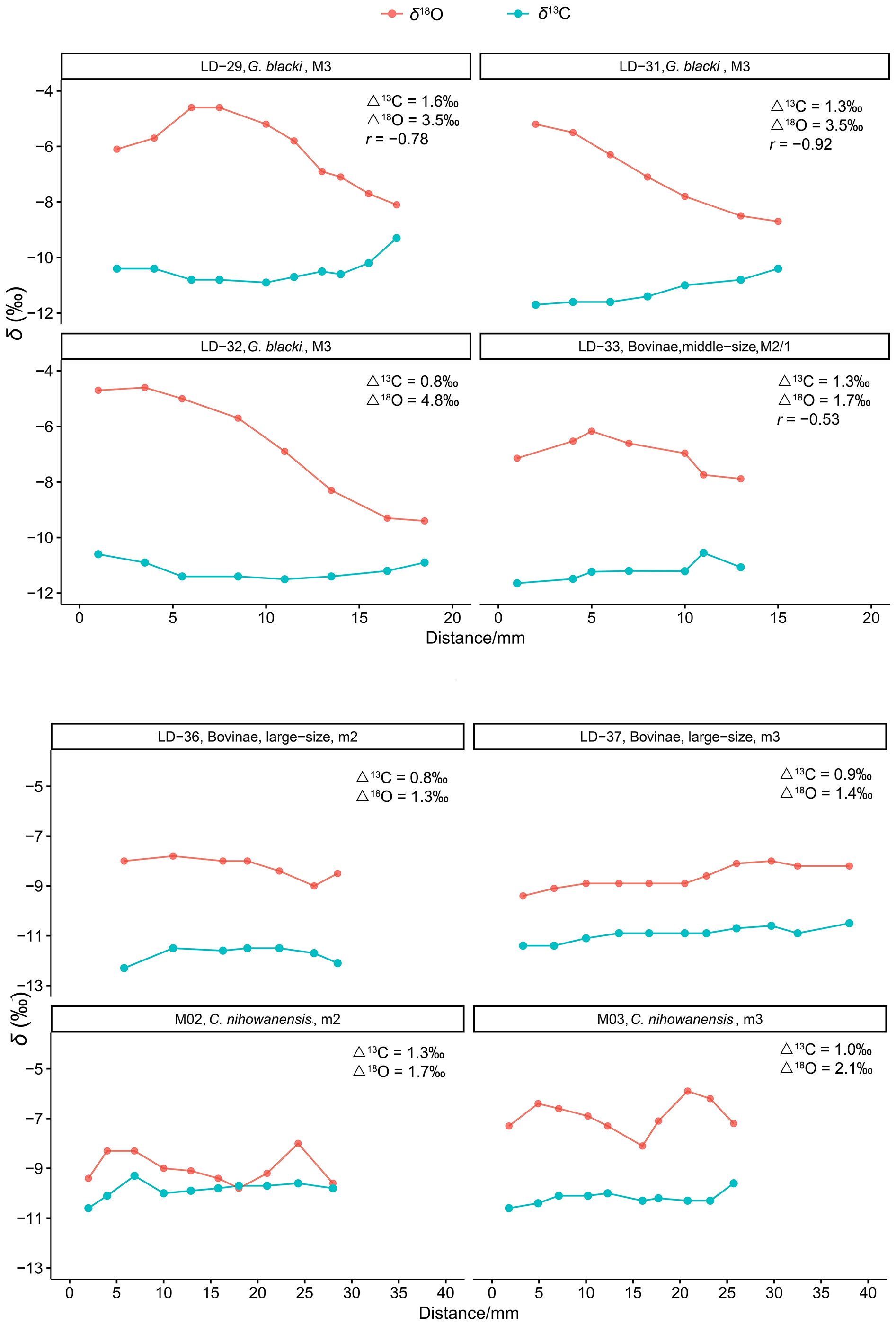
Figure 2 Serial isotopic profiles of the fossil mammals in this study. (Δ13C and Δ18O are the ranges of the intra-tooth δ13C and δ18O values).
The intra-tooth δ18O ranges of three G. blacki specimens were 3.5‰, 3.5‰, and 4.8‰, exhibiting greater variability compared to those of the Bovinae (1.7‰, 1.3‰, and 1.4‰) and C. nihowanensis (1.7‰, 2.1‰) specimens. The intra-tooth δ18O ranges of the C. nihowanensis specimens were slightly larger than those of the Bovinae specimens in this study and equid specimens (0.7‰, 1.4‰, 1.5‰, and 2.3‰) in Ma et al. (2023). Regular sinusoidal patterns were observed in the serial δ18O profiles of the C. nihowanensis specimens (Figure 2). This suggested that C. nihowanensis specimens possibly obtain fewer water resources from the longstanding water bodies, unlike the large Bovinae and equids (Biasatti et al., 2010; Ma et al., 2023). The distinct water resources of the C. nihowanensis specimens, compared to the Bovinae and equids, previously inferred from their higher δ18O values (Table 1; Supplementary Table S3), were consistent with this finding inferred from the different serial isotopic profiles. Alternatively, C. nihowanensis specimens might be non-migratory and thus could record the δ18O signals of local environments for more than one year (Figure 2).
Furthermore, an inverse relationship between the intra-tooth δ13C and δ18O values were observed for two G. blacki specimens (LD-29, 31; r =−0.78, −0.92) and one middle-sized Bovinae specimen (LD-33, r =−0.53).
In Supplementary Figure S1, we plotted the serial isotope profiles of two modern goats from the different regions in the Linxia Basin and its vicinity with varying altitudes. The average δ13C values of these two samples were −10.1± 2.8‰ (n=6) and −11.9± 0.9‰ (n=5), respectively; and their mean δ18O values were −5.2± 1.4‰ and −7.1± 1.9‰. The intra-tooth range of δ13C values for one goat specimen was higher, and its serial δ13C values ranged from −13.4‰ to −6.3‰, clearly indicating seasonal C4 food resources. The intra-tooth δ18O ranges of these two goats were 4.5‰ and 3.6‰, slightly higher than those of three fossil G. blacki specimens. Additionally, inverse relationships between δ13C and δ18O values were observed for both goat specimens (r = −0.83, −0.86).
The isotopic results of the modern goats indicated the occurrence of East Asia Summer Monsoon (EASM) and the presence of seasonal C4 plants. For both specimens, the peaks of δ13C values indicated the ingestion of C4 plants during the summer, and their corresponding δ18O values were the lowest. In a monsoonal region, the “amount effect” affects seasonal δ18O values of precipitation, preferentially removing 18O from vapor, resulting in lower δ18O values in summer (Dansgaard, 1964; Rozanski et al., 1993). High precipitation and warm temperatures favor C4 plants, which are naturally more abundant in summer. Therefore, an inverse relationship between δ13C and δ18O values is expected (Higgins and MacFadden, 2004; Wang et al., 2008b; Biasatti et al., 2010; Yang et al., 2012; Zhang et al., 2012; Ciner et al., 2015).
In Figure 2 and Supplementary Figure S1, the serial isotopic profiles of the two modern goats were similar to those of a part of fossil teeth in this study, especially the G. blacki specimens. Through these modern references, this study provides evidence once again that the EASM occurred in the Linxia Basin during the Early Pleistocene (Biasatti et al., 2010). Furthermore, it appears that non-obligate drinkers offer better indicators of seasonal variations in local environments than large obligate drinkers (Roberts et al., 2018; Pederzani and Britton, 2019; Hodgkins et al., 2020).
Based on this and previous studies (Wang and Deng, 2005; Biasatti et al., 2010; Ma et al., 2023), the paleoenvironment in the Linxia Basin, as inferred from mammalian carbon isotopes during the Early Pleistocene was primarily characterized by C3 plants, with C4 plants likely representing a minor component that was seasonally consumed by the studied mammals. This finding aligns with the isotopic results of the carbonates from fluvial and lacustrine sediments (Dettman et al., 2003; Hough et al., 2011). The sub-humid climate and the occurrence of EASM are further supported by previous studies, including stable isotope analysis (Biasatti et al., 2010), fossil assemblage analysis (Qiu et al., 2002; Wang, 2005; Xue et al., 2006), and sedimentary analysis (Zan et al., 2016).
5 Discussion
5.1 Foraging ecologies and environmental contexts of C. nihowanensis in Early Pleistocene northern China
Studying the foraging ecologies of C. nihowanensis in northern China from west to east can provide valuable insights into the relationship between their ecological behaviors and paleoenvironments during the Early Pleistocene. This study provides a comprehensive insight into the paleoecology of the earliest C. nihowanensis in northwestern China, situated on the edge of the Tibetan Plateau. Within the Linxia Basin, C. nihowanensis specimens were likely a mixed feeder inhabiting diverse habitats, as indicated by their wide distribution of the δ13C values and intermediate δ18O values compared to other coexisted mammals. Meanwhile, recent stable isotopic studies on mammalian faunas in the Nihewan Basin aiming to explore the paleoenvironmental settings of early humans can serve as a strong evidence for the paleoecology of C. nihowanensis in eastern China (Xu et al., 2021; Xu et al., 2023). Therefore, it is a great chance to compile all these data and explore the paleoecological changes of the C. nihowanensis (Figure 3; Supplementary Table S4). Besides, the same pretreatment methods of the stable isotopic analysis for these two faunas in the same laboratory could guarantee the validity of the cross comparison. Considering the potential bias in δ18O values stemmed from variations in phosphoric acid reaction temperatures (Passey et al., 2007) during the isotopic measurements conducted in different laboratories, the isotopic data from Wang and Deng (2005) as well as Biasatti et al. (2010, 2018) were deliberately not included in Figure 3. It is worth noting, however, that the intra-tooth isotopic ranges from these studies have been collated and are presented in Figure 4 (Supplementary Table S5). The altitude of the Linxia and Nihewan basins during the Early Pleistocene were already situated at their current elevations and therefore, δ13Cdiet values were corrected with the modern altitudes of 2248 m and ~940 m (Deng et al., 2013; Pei et al., 2019), respectively. δ13Cdiet-adj-alt were corrected using the formula described in section 3.4. This correction was applied at the Longdan and Shanshenmiaozui sites, where the ungulates’ diets predominantly consisted of almost pure C3 plants. However, in light of the significant components of the C4 plants in the diet of these ungulates, this correction was not applied in other three sites during ca. 1.2–1.1 Ma in the Nihewan Basin.
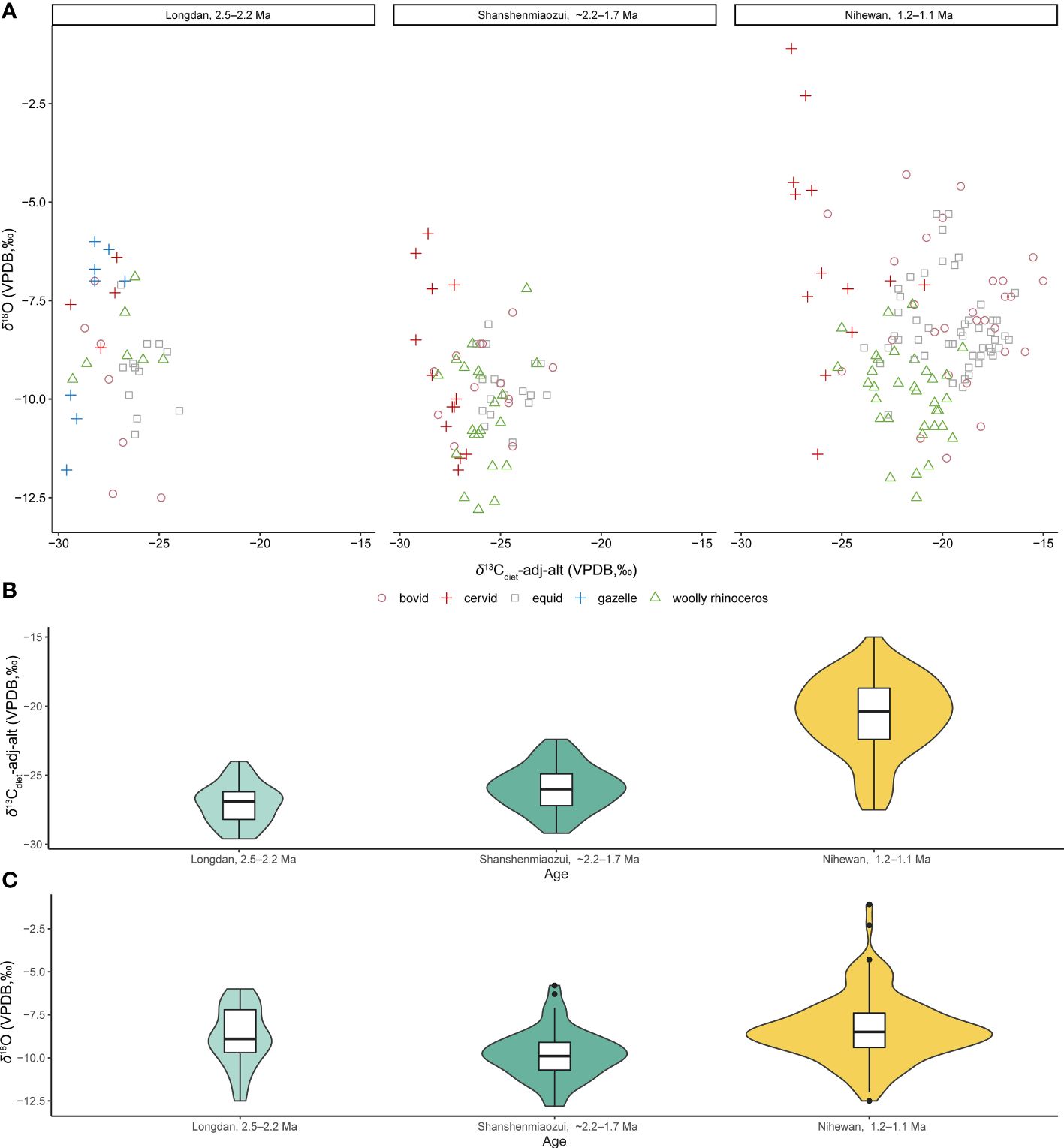
Figure 3 δ13Cdiet-adj-alt and δ18O values of the Longdan fauna and Nihewan fauna (~2.2–1.7 Ma: Shanshenmiaozu; ca. 1.2–1.1 Ma: Madigou, Feiliang, and Donggutuo) (Xu et al., 2021, 2023; Ma et al., 2023; this study) (Supplementary Table S4). (A) Scatter plot of δ13Cdiet-adj-alt and δ18O values of different taxa. (B) Violin plot and boxplot of δ13Cdiet-adj-alt values of all the mammalian species. (C) Violin plot and boxplot of δ18O values of all the mammalian species. (These data consist of bulk samples and the mean values of each serial sample. For panels B and C, the width of the “violin” at a particular value reflects the data density at that point. Within the boxplot, the lower hinge, median line, and upper hinge denote the 25th percentile, median, and the 75th percentile, respectively. The lower whisker extends to the smallest observation at or above the first quartile (Q1) minus 1.5 times the Interquartile Range (IQR), while the upper whisker extends to the largest observation at or below the third quartile (Q3) plus 1.5 times the IQR. The potential outliers in the dataset are marked by separate points).
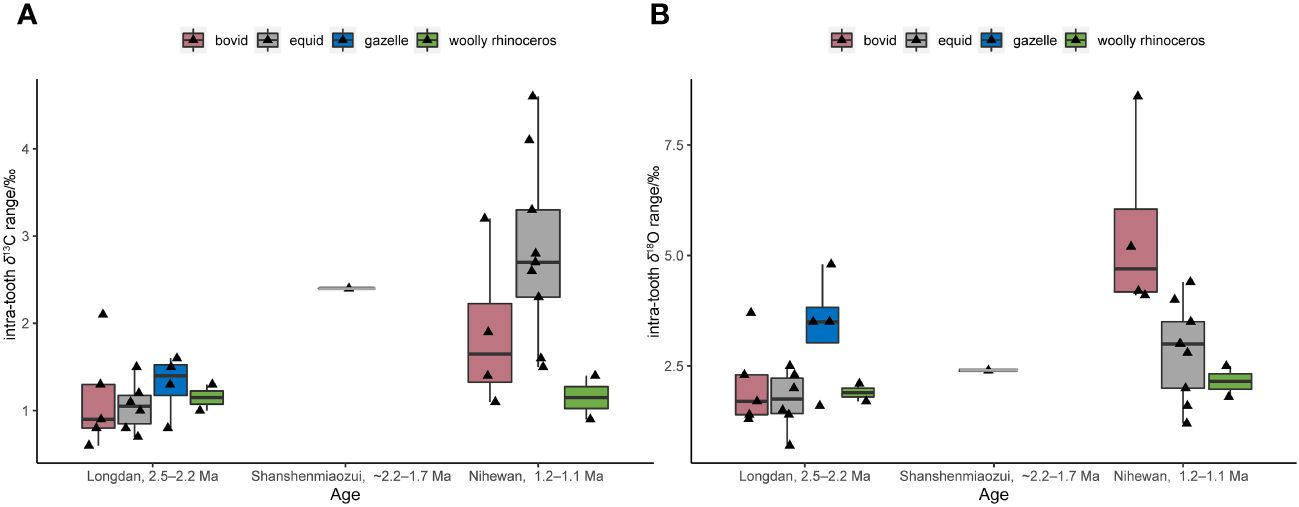
Figure 4 Intra-tooth δ13C (A) and δ18O ranges (B) of the serial samples in the Linxia Basin (Longdan) and Nihewan Basin (Shanshenmiaozu, ~2.2–1.7 Ma; Madigou, Feiliang, and Donggutuo are ca. 1.2–1.1 Ma) (Biasatti et al., 2010; Xu et al., 2021, 2023; Ma et al., 2023; this study) (Supplementary Table S5). (The boxplot conveys the same meaning as Figure 3, but without annotating the outliers to avoid redundancy with the original data points represented by the jitter scatter plot).
Among the four sites in the Nihewan Basin, Shanshenmiaozui has yielded the richest mammalian fossils. Based on the analysis of the faunal assemblage, the age of the Shanshenmiaozui fauna likely corresponds closely with the classic Nihewan fauna during the early Early Pleistocene, with an approximate age of 2.2–1.7 Ma (Tong et al., 2021). The other three sites–Madigou, Donggutuo, and Feiliang–are classified as Early Paleolithic, with the age of ca. 1.2–1.1 Ma, late Early Pleistocene (Wang et al., 2005; Deng et al., 2007; Pei et al., 2019).
In Figure 3, the δ13Cdiet-adj-alt values of the Shanshenmiaozui fauna (−25.9± 1.6‰, n=65) were higher than those of the Longdan fauna (−27.1± 1.4‰, n=39) (p-value of Mann-Whitney U test: 0.0005) (Ma et al., 2023; Xu et al., 2023; this study). However, such variation may not be directly linked to the difference in landscape between these two sites (Negash and Barr, 2023). The δ18O values of the Shanshenmiaozui fauna (−9.8± 1.4‰, n=65) were slightly lower than those of the Longdan fauna (−8.8± 1.7‰, n=39) (p-value of Mann-Whitney U test: 0.001), possibly indicating a wetter and/or more humid environment in the Nihewan Basin during the early Early Pleistocene (Ma et al., 2023; Xu et al., 2023; this study).
The intra-tooth δ13C and δ18O ranges of the single equid specimen in Shanshenmiaozui were higher than those of the Longdan equid specimens (Figure 4) (Biasatti et al., 2010; Ma et al., 2023; Xu et al., 2023; this study). This might suggest a more pronounced climatic seasonality in the Nihewan Basin compared to the Linxia Basin, despite the limited serial sample amount in Shanshenmiaozui. Nonetheless, it seems that C4 grasses may not have become a significant component of the local ecosystems in the Nihewan Basin during this period.
In the Shanshenmiaozui fauna, C. nihowanensis and bovids exhibited intermediate δ13Cdiet-adj-alt values, which were lower than those of equids but higher than cervid ones (Figure 3; Supplementary Table S6) (Xu et al., 2023). This indicated that C. nihowanensis and bovid specimens in Shanshenmiaozui likely inhabited mixed grasslands and woodlands. Statistical analyses displayed no differences in the δ18O values among these groups (Supplementary Table S7). However, δ18O values were lowest for C. nihowanensis among all the coexisting mammals (Figure 3), possibly indicating that these specimens obtained abundant meteoric water and possibly inhabit areas close to lakes and/or rivers (Xu et al., 2023).
Until the late Early Pleistocene, the mean δ13Cdiet-adj-alt and δ18O values of the three sites during ca. 1.2–1.1 Ma were higher than those of the Shanshenmiaozui fauna (p-values of Mann-Whitney U tests for δ13C and δ18O: 0.0005, 0.0001) (Figure 3) (Xu et al., 2021; Xu et al., 2023). This indicated that the C4 vegetation became significant in the Nihewan Basin during the late Early Pleistocene. The intra-tooth ranges in δ13C and δ18O values of the equid and bovid specimens at these three sites were greater than those of the Longdan and Shanshenmiaozui specimens (Figure 4; Supplementary Table S5) (Biasatti et al., 2010; Xu et al., 2021; Ma et al., 2023; Xu et al., 2023; this study), possibly indicating seasonal ingestion of a substantial amount of C4 plants in their diets and more pronounced seasonal fluctuations in precipitation. An inverse relationship between δ13C and δ18O values was observed in some serial equid and bovid specimens in Donggutuo and Feiliang sites (Supplementary Table S5) (Xu et al., 2023), which was the signal of the EASM as aforementioned. Therefore, the paleoenvironments in the Nihewan Basin during the late Early Pleistocene were possibly varied and experienced pronounced seasonality under the effect of intensive EASM and the winter monsoon (Xu et al., 2021; Xu et al., 2023).
The δ13Cdiet-adj-alt values of the C. nihowanensis specimens during ca. 1.2–1.1 Ma were also lower than those of equid and bovid specimens but higher than cervid ones (Figure 3; Supplementary Table S8) (Xu et al., 2021; Xu et al., 2023), indicating that C. nihowanensis specimens fed on mixed C3/C4 vegetation, and consumed less C4 grasses than equids and bovids. Furthermore, C. nihowanensis specimens exhibited the lowest δ18O values (Figure 3; Supplementary Table S9), and the intra-tooth ranges in δ13C and δ18O values of C. nihowanensis were found to be smaller than those of the equids and bovids (Figure 4; Supplementary Table S5) (Xu et al., 2021; Xu et al., 2023). This possibly indicated that C. nihowanensis occupied relatively close and well-watered environments which experienced fewer seasonal changes. Therefore, C. nihowanensis in the Nihewan Basin during the late Early Pleistocene was likely a mixed feeder and showed a preference for close environments (Xu et al., 2021; Xu et al., 2023).
During the early Early Pleistocene, the paleoenvironments in the Linxia and Nihewan basins were primarily dominated by C3 vegetation, with the latter likely exhibited a stronger EASM compared to the Linxia Basin. Previous studies found that C4 signals were more abundant in the Linxia Basin at ~1.2 Ma (Wang and Deng, 2005; Biasatti et al., 2010). The increasing prevalence of C4 plants and a pronounced EASM in the Linxia and Nihewan basins around 1.2–1.1 Ma potentially aligns with the widespread aridification in northern China (Da et al., 2015; Zhou et al., 2018). Against this backdrop, C. nihowanensis owned a notable dietary flexibility and were able to thrive in a variety of environments across northern China from west to east throughout the Early Pleistocene. Such ecological adaptability of C. nihowanensis is presumed to have been influenced by the intricate terrain of Early Pleistocene East Asia and its diverse environments, which were situated along the migration path of the woolly rhinoceroses from the Tibetan Plateau to northern Eurasia.
5.2 Ecological adaptation of Coelodonta and coexisting mammals
After thoroughly examining the isotopic paleoecology of C. nihowanensis in Early Pleistocene East Asia, it is imperative to delve deeper into the paleoecology of its ancestral and derived species to capture the paleoecological evolution of Coelodonta. We complied all δ13Cdiet-adj-alt values of the Coelodonta genus and other coexisting mammals in East Asia from the mid-Pliocene to Late Pleistocene in Figure 5 (Supplementary Table S4). During the mid-Pliocene, it was estimated that the elevation of the Zanda Basin was similar to its modern altitude, approximately 4,000 meters asl (Deng et al., 2012). Given that the modern altitudes of these Late Pleistocene sites were around 100–200 meters, it is assumed that the altitude effect could be disregarded in this context.
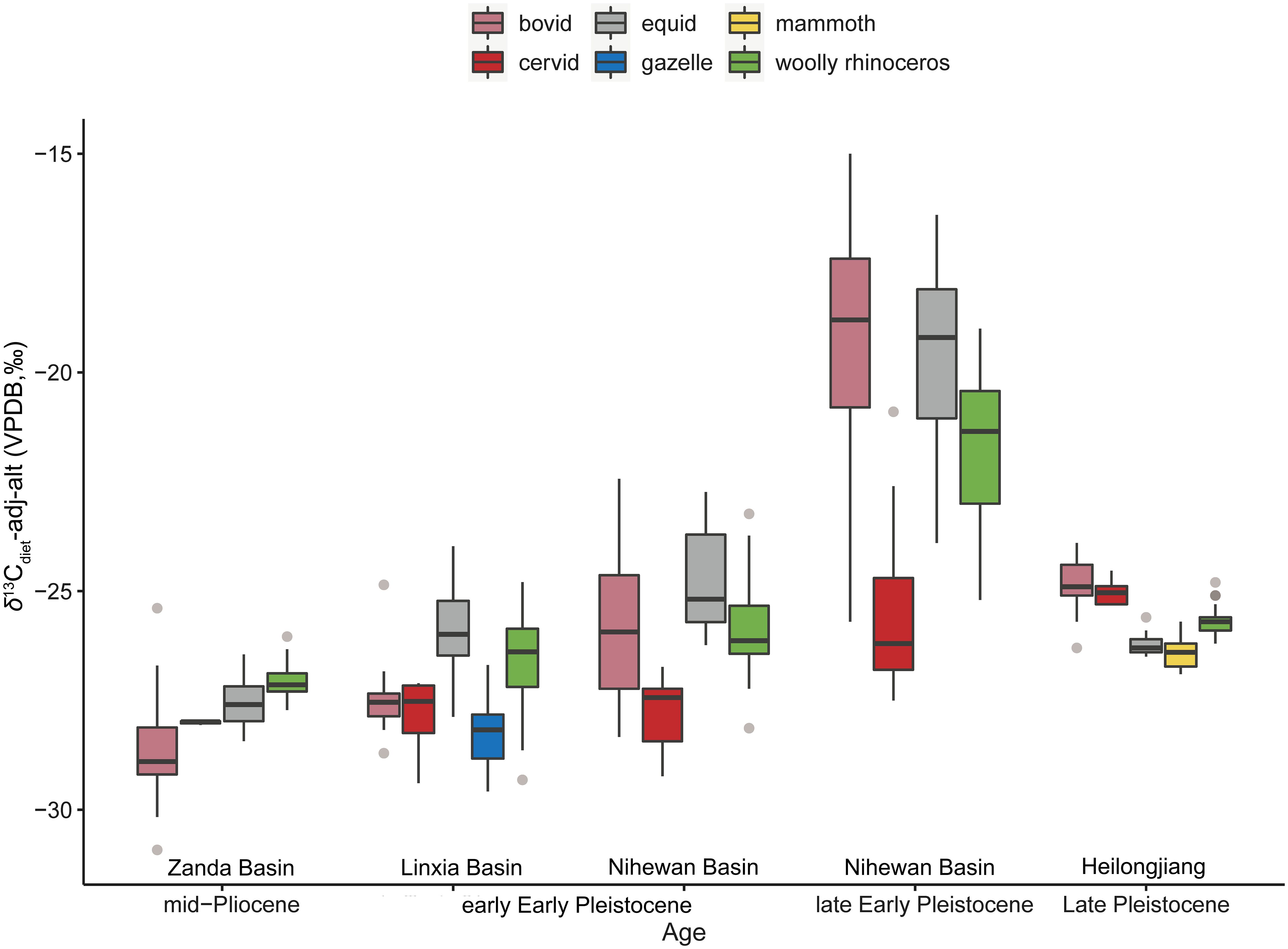
Figure 5 δ13Cdiet-adj-alt values of the Coelodonta genus from the mid-Pliocene to Late Pleistocene (Wang and Deng, 2005; Wang Y. et al., 2013; Ma et al., 2017; Biasatti et al., 2018; Ma et al., 2021; Xu et al., 2021; Ma et al., 2023; Xu et al., 2023; this study) (the box plot conveys the same meaning as Figure 3).
During the mid-Pliocene, the δ13Cdiet-adj-alt values of C. thibetana in the Zanda Basin ranged narrowly between −27.7‰ to −26.0‰ (n=22), with their mean δ13Cdiet-adj-alt (−27.0± 0.4‰) being higher than those of the bovid (−28.6± 1.3‰, n=19), equid (−27.6± 0.5‰, n=30), and cervid (−28.0± 0.1‰, n=3) specimens (Supplementary Table S10; Figure 5) (Wang Y. et al., 2013). Additionally, both carbon and oxygen isotopes of the C. thibetana specimens showed lower standard deviations than those of the bovids and equids (Figure 5; Wang Y. et al., 2013). This suggested that the ancestral C. thibetana primarily fed on C3 grasses and occupied a confined ecological niche in open environments at the high-altitude Zanda Basin. C. thibetana occupies the most basal position of the Coelodonta lineage and was considered the earliest representative of this genus (Deng et al., 2011). The morphological traits of the C. thibetana, such as the highly elongated skull, large and roughened nasal horn base, and high tooth crowns with rough enamel, are indicative of an adaptation to efficiently graze in cold and arid environments. These were in consistent with its foraging ecology inferred from stable isotopes.
C. thibetana moved down from the Tibetan Plateau toward northeastern Linxia Basin (~2,500 m asl) at the initiation of the Quaternary and evolved as C. nihowanensis. The average δ13Cdiet-adj-alt value of the Longdan fauna (−26.9± 1.4‰, n=55) (Wang and Deng, 2005; Biasatti et al., 2018; Ma et al., 2023; this study) was higher than that of the Zanda fauna (−27.7± 1.0‰, n=74) (p-value of Mann-Whitney U test: 0.0008) (Wang Y. et al., 2013), possibly indicating a drier/warmer climate in the Early Pleistocene Linxia Basin. The broader range of δ13Cdiet-adj-alt values observed in the C. nihowanensis specimens in the Linxia Basin (Biasatti et al., 2018; this study) compared to coexisting mammals, in contrast to the narrower range of the δ13Cdiet-adj-alt values seen in the ancestral C. thibetana in the Zanda fauna, suggested that C. nihowanensis diversified its ecological niche during this period. In the Zanda and Longdan faunas, both woolly rhinoceroses and equids had higher δ13Cdiet-adj-alt values than those of artiodactyls (Figure 5) (Wang Y. et al., 2013), suggesting a possible ecological niche partitioning between artiodactyls and perissodactyls.
In Section 5.1, a thorough discussion has been conducted on the paleoenvironmental and paleoclimatic changes in the Nihewan Basin during the Early Pleistocene. In the Shanshenmiaozui fauna, equids occupied open grasslands and exhibited the highest δ13Cdiet-adj-alt values (−24.8± 1.1‰, n=18) among all the mammalian species (Supplementary Table S6) (Xu et al., 2023). Similar δ13Cdiet-adj-alt values were observed for woolly rhinoceroses (−25.9± 1.2‰, n=21) and bovids (−25.7± 1.7‰, n=13) in this fauna (Supplementary Table S6), indicating similar habitat preferences for both species (Xu et al., 2023). The lowest δ13Cdiet-adj-alt values (−27.8± 0.8‰, n=13) of the cervid specimens reflected a preference for browsing in a more close environment (Supplementary Table S6) (Xu et al., 2023). Therefore, C. nihowanensis and bovids in Shanshenmiaozui were possibly mixed feeders and likely inhabited relatively open environments in Shanshenmiaozui. These ecological niches within the Shanshenmiaozui fauna inferred from the δ13Cdiet-adj-alt values were similar to the pattern observed in the Longdan fauna.
In the Feiliang, Madigou, and Donggutuo sites, bovids and equids exhibited much higher δ13Cdiet-adj-alt values (−19.3± 2.6‰, n=29; −19.6± 1.9‰, n=51) than those of the woolly rhinoceroses (−21.7± 1.6‰, n=34) and cervids (−25.6± 2.0‰, n=13) (refer to Supplementary Table S8) (Xu et al., 2021; Xu et al., 2023). This suggested that the former consumed a greater amount of C4 grasses and inhabited more open environments compared to the latter during the late Early Pleistocene. C. nihowanensis likely had a mixed diet and favored a relatively close habitat during ca. 1.2–1.1 Ma. This contrasted slightly with the preference for open environments observed in earlier C. nihowanensis specimens from Shanshenmiaozui within the same region. Consequently, it seems that C. nihowanensis in the Nihewan Basin displayed varying ecological preferences during different periods of the Early Pleistocene.
The ecological niche partitioning among these mammalian taxa during the late Early Pleistocene differed from the pattern observed in previous faunas, indicating notable ecological shifts among these mammalian taxa in eastern China. Throughout the mid-Pliocene to late Early Pleistocene, equids consistently favored open environments, reflecting a grazing preference similar to their modern counterparts (Ransom and Kaczensky, 2016). Within the Nihewan Basin, bovids exhibited a highly diverse foraging ecology, gradually expanding into open environments compared to the Zanda and Linxia basins (Xu et al., 2021; Xu et al., 2023). However, the habitats of the C. nihowanensis slightly shifted towards more close environments in the Nihewan Basin (Xu et al., 2021; Xu et al., 2023). This suggested distinct foraging strategies between bovids and woolly rhinoceroses in response to changing paleoenvironments.
C. nihowanensis is estimated to have a larger body size (approximately 1700 kg according to the NOW database1) compared to the ancestral C. thibetana (~1470 kg1), and its morphological traits were also considered as a grazing adaptation in cold and dry environments. However, the foraging ecologies of C. nihowanensis were more flexible and even displayed a preference for close environments. Additionally, the limb bones of C. nihowanensis are more slender than those of the derived C. tologoijensis, indicating a better running ability (Deng, 2008). However, the isotopic results of the C. nihowanensis specimens from both Linxia and Nihewan basins likely exhibited a potential of non-migratory behaviors as aforementioned. This highlights the distinction between individual behavioral selection and population dispersal as well as evolutionary history.
C. tologoijensis (~2000 kg1) has a remarkably larger body size than the primary C. nihowanensis, and its paleoecology was rarely explored. According to the paleoenvironmental records of the sites in Asia which preserved C. tologoijensis fossils, Kahlke and Lacombat (2008) presumed that C. tologoijensis possibly inhabited meadow to forest steppes, similar to those found in northern Mongolia today.
C. antiquitatis first occurred during the late Middle Pleistocene and thrived in northern Eurasia during the Late Pleistocene as an emblematic member in the Mammuthus-Coelodonta Faunal Complex, and its paleoecology was intensively explored. C. antiquitatis was graviportal and has a massive body size (~2900 kg1), as a highly progressive species of Coelodonta (Boeskorov, 2001; Stuart and Lister, 2012). A series of anatomical features observed in C. antiquitatis, including wide lips, low posture of the head, long nasal horn, high tooth crown, long hair, and dense underwool, all indicating an adaptation for grazing in dry and frosty environments (Kahlke and Lacombat, 2008; Boeskorov et al., 2011b; Boeskorov, 2012).
The increasing number of paleoecological studies using multiple methods has revealed that C. antiquitatis from the northern Eurasia was a typical grazer with a highly abrasive diet, including dental microwear and mesowear analyses (Rivals et al., 2010; Rivals and Lister, 2016; Rivals and Álvarez-Lao, 2018; Pushkina et al., 2020), stable isotope analysis (e.g. Bocherens et al., 1991; Bocherens et al., 1997; Pushkina et al., 2014; Schwartz-Narbonne et al., 2019), and the examination of plant remains from gastro-intestinal content (Boeskorov et al., 2011a; Stefaniak et al., 2021) and teeth (van Geel et al., 2019; Stefaniak et al., 2021). Nonetheless, some studies have indicated a more mixed diet, signifying dietary plasticity and seasonality of C. antiquitatis (Rivals et al., 2010; Tiunov and Kirillova, 2010; Rivals and Lister, 2016; Rey-Iglesia et al., 2021).
The stable carbon and nitrogen isotope analyses of the Mammuthus-Coelodonta Faunal Complex in Heilongjiang suggested that C. antiquitatis may have been a grazer in East Asia, while also exhibiting dietary flexibility (as indicated by their intermediate δ13C and relatively high δ15N values, see Supplementary Tables S4, S11, S12; the isotopic pattern of the plants on the mammoth steppe was different from other biomes discussed here) (Ma et al., 2017; Ma et al., 2021). Within this fauna, delineation of ecological niche using carbon and nitrogen isotopic signatures roughly suggested a grazing ecology for mammoths, woolly rhinoceroses, and bovids, while equid specimens appear to have consumed more shrub or tree leaves, and cervid specimens likely ingested lichens, mosses, and leaves (Ma et al., 2017; Ma et al., 2021). This suggests that various taxa exhibited distinct ecological strategies when confronted with a completely distinct paleoenvironments during the Late Pleistocene.
The abundance and wide distribution of C. antiquitatis in Late Pleistocene East Asia have been well-documented (Tang et al., 2003; Li and Dong, 2007; Dong et al., 2014; Chen et al., 2016; Li et al., 2018). C. antiquitatis coexisted with woolly mammoths in northeastern China and the straight-tusk elephant Palaeoloxodon in warmer zones, with the southernmost boundary at 33° latitude (Tong, 2004; Tong et al., 2008; Dong et al., 2014; Wang et al., 2021). Coelodonta-Palaeoloxodon sympatry also occurred in England (Schreve, 2001, 2019). The faunal mixture and the wide distribution of C. antiquitatis may be linked to glacial/interglacial cycles, yet they also suggest the high ecological adaptability of C. antiquitatis, enabling this species to flourish in both cold and temperate environments.
This study conducted a comprehensive review of the paleoecological and evolutionary history of the woolly rhinoceroses from a spatiotemporal perspective. The ancestral C. thibetana and the terminating C. antiquitatis were more likely grazers that inhabited the high-altitude Tibetan Plateau during the mid-Pliocene and the middle to high latitude Eurasia during the Late Pleistocene. In contrast, C. nihowanensis, an intermediate form of the woolly rhinoceroses, exhibited flexible foraging ecologies across varied environments from west to east in northern China. The wide distribution of C. antiquitatis in temperate regions also indicates its high environmental tolerance. As an iconic cold-adapted mammal, Coelodonta adjusted its ecological strategies upon departing the Tibetan Plateau, ultimately dispersing from East Asia and colonizing middle to high latitude Eurasia. Further exploration of the paleoecology of the Middle Pleistocene C. tologoijensis will enhance our understanding of the complete ecological evolution of Coelodonta. While this paper reconstructed the paleoecology of the genus Coelodonta from an isotopic perspective, it is evident that further multidisciplinary investigations including systematic stratigraphy analysis, microwear and mesowear analyses, ecomorphological analysis, and locomotive behavior analysis are required to gain a more comprehensive understanding of the paleoecology of the ancestors of the woolly rhinoceroses.
Data availability statement
The original contributions presented in the study are included in the article/Supplementary Material. Further inquiries can be directed to the corresponding author.
Author contributions
JM: Conceptualization, Data curation, Funding acquisition, Investigation, Methodology, Writing – original draft, Writing – review & editing. SW: Project administration, Resources, Writing – original draft, Writing – review & editing. TD: Conceptualization, Investigation, Resources, Writing – original draft, Writing – review & editing.
Funding
The author(s) declare financial support was received for the research, authorship, and/or publication of this article. This work was supported by State Key Laboratory of Palaeobiology and Stratigraphy (Nanjing Institute of Geology and Palaeontology, CAS) (No. 223119), the National Natural Science Foundation in China (No. 42002005), Award of Youth Innovation Promotion Association (No. 2022070), and National Key Research and Development Program of China (No. 2023YFF0804501, 2022YFF0903800).
Acknowledgments
We appreciate BY Sun, QQ Shi, and Y Chen for identifying the specimens and engaging in useful discussions. We extend our gratitude to L. Zhang from the Hezheng Paleontological Museum for assisting in collecting fossil specimens, and to M.Q. Ma (the first author's father) for gathering modern samples. We thank H.W. Li and H.Y. Rao for their help in the lab. We thank two reviewers (Maciej Tomasz Krajcarz and Naoto Handa) and the Editor Florent Rivals for valuable suggestions, which have highly improved this manuscript. We would like to thank ChatGPT 3.5 for its valuable inputs and assistance for improving the language and checking grammar of this manuscript.
Conflict of interest
The authors declare that the research was conducted in the absence of any commercial or financial relationships that could be construed as a potential conflict of interest.
Publisher’s note
All claims expressed in this article are solely those of the authors and do not necessarily represent those of their affiliated organizations, or those of the publisher, the editors and the reviewers. Any product that may be evaluated in this article, or claim that may be made by its manufacturer, is not guaranteed or endorsed by the publisher.
Supplementary material
The Supplementary Material for this article can be found online at: https://www.frontiersin.org/articles/10.3389/fevo.2024.1377000/full#supplementary-material
Footnotes
- ^ The NOW Community. New and Old Worlds Database of Fossil Mammals (NOW). Licensed under CC BY 4.0. https://nowdatabase.org/now/database/.
References
Ambrose S. H., Norr L. (1993). “Experimental evidence for the relationship of the carbon isotope ratios of whole diet and dietary protein to those of bone collagen and carbonate,” in Prehistoric Human Bone. Eds. Lambert J. B., Grupe G. (Springer, Berlin, Heidelberg), 1–37.
Balasse M. (2002). Reconstructing dietary and environmental history from enamel isotopic analysis: time resolution of intra-tooth sequential sampling. Int. J. Osteoarchaeol. 12, 155–165. doi: 10.1002/oa.601
Bender M. M. (1968). Mass spectrometric studies of carbon 13 variations in corn and other grasses. Radiocarbon 10, 468–472. doi: 10.1017/S0033822200011103
Biasatti D., Wang Y., Deng T. (2010). Strengthening of the East Asian summer monsoon revealed by a shift in seasonal patterns in diet and climate after 2–3Ma in northwest China. Palaeogeogr. Palaeoclimatol. Palaeoecol. 297, 12–25. doi: 10.1016/j.palaeo.2010.07.005
Biasatti D., Wang Y., Deng T. (2018). Paleoecology of Cenozoic rhinos from northwest China: a stable isotope perspective. Vertebr. Palasiat. 56, 45–68. doi: 10.19615/j.cnki.1000-3118.170519
Blumenthal S. A., Cerling T. E., Chritz K. L., Bromage T. G., Kozdon R., Valley J. W. (2014). Stable isotope time-series in mammalian teeth: In situ δ18O from the innermost enamel layer. Geochim. Cosmochim. Acta 124, 223–236. doi: 10.1016/j.gca.2013.09.032
Blumenthal S. A., Cerling T. E., Smiley T. M., Badgley C. E., Plummer T. W. (2019). Isotopic records of climate seasonality in equid teeth. Geochim. Cosmochim. Acta 260, 329–348. doi: 10.1016/j.gca.2019.06.037
Bocherens H., Billiou D., Patou-Mathis M., Bonjean D., Otte M., Mariotti A. (1997). Paleobiological implications of the isotopic signatures (13C, 15N) of fossil mammal collagen in Scladina Cave (Sclayn, Belgium). Quat. Res. 48, 370–380. doi: 10.1006/qres.1997.1927
Bocherens H., Fizet M., Mariotti A., Lange-Badre B., Vandermeersch B., Borel J. P., et al. (1991). Isotopic biogeochemistry (13C,15N) of fossil vertebrate collagen: application to the study of a past food web including Neandertal man. J. Hum. Evol. 20, 481–492. doi: 10.1016/0047-2484(91)90021-M
Boeskorov G. G. (2001). Woolly rhino (Coelodonta antiquitatis) distribution in Northeast Asia. Deinsea 8, 15–20.
Boeskorov G. G. (2012). Some specific morphological and ecological features of the fossil woolly rhinoceros (Coelodonta antiquitatis Blumenbach 1799). Biol. Bull. 39, 692–707. doi: 10.1134/S106235901208002X
Boeskorov G. G., Bakulina N. T., Davydov S. P., Shchelchkova M. V., Solomonov N. G. (2011a). Study of pollen and spores from the stomach of a fossil woolly rhinoceros found in the lower reaches of the Kolyma river. Dokl. Biol. Sci. 436, 23–25. doi: 10.1134/S0012496611010017
Boeskorov G. G., Lazarev P. A., Sher A. V., Davydov S. P., Bakulina N. T., Shchelchkova M. V., et al. (2011b). Woolly rhino discovery in the lower Kolyma River. Quat. Sci. Rev. 30, 2262–2272. doi: 10.1016/j.quascirev.2011.02.010
Bryant J. D., Froelich P. N. (1995). A model of oxygen isotope fractionation in body water of large mammals. Geochim. Cosmochim. Acta 59, 4523–4537. doi: 10.1016/0016-7037(95)00250-4
Cerling T. E., Bernasconi S. M., Hofstetter L. S., Jaggi M., Wyss F., Rudolf von Rohr C., et al. (2021). CH4/CO2 ratios and carbon isotope enrichment between diet and breath in herbivorous mammals. Front. Ecol. Evol. 9, 638568. doi: 10.3389/fevo.2021.638568
Cerling T. E., Harris J. M. (1999). Carbon isotope fractionation between diet and bioapatite in ungulate mammals and implications for ecological and paleoecological studies. Oecologia 120, 347–363. doi: 10.1007/s004420050868
Cerling T. E., Harris J. M., MacFadden B. J., Leakey M. G., Quade J., Eisenmann V., et al. (1997). Global vegetation change through the Miocene/Pliocene boundary. Nature 389, 153–158. doi: 10.1038/38229
Chen J., Yin Y. Q., li T., Jin L. Y. (2016). The Mammuthus-Coelodonta fauna from Dabusu national key fossil locality, Jilin Province. Geol. Bull. China 35, 872–878. doi: 10.3969/j.issn.1671-2552.2016.06.004
Chen X., Yang M., Zhao H. (2023). Early Pleistocene mammal fossils from Niujuangou locality, Nihewan Basin, Hebei Province. Quat. Sci. 43, 763–776. doi: 10.11928/j.issn.1001-7410.2023.03.07
Chen X., Zhao H., Zhang B., Tong H. (2017). Report on the excavation of the early Pleistocene Shigou site B in Nihewan Basin, northern China. Quat. Sci. 37, 895–907. doi: 10.11928/j.issn.1001-7410.2017.04.24
Ciner B., Wang Y., Deng T., Flynn L., Hou S., Wu W. (2015). Stable carbon and oxygen isotopic evidence for Late Cenozoic environmental change in Northern China. Palaeogeogr. Palaeoclimatol. Palaeoecol. 440, 750–762. doi: 10.1016/j.palaeo.2015.10.009
Da J., Zhang Y. G., Wang H., Balsam W., Ji J. (2015). An Early Pleistocene atmospheric CO2 record based on pedogenic carbonate from the Chinese loess deposits. Earth Planet. Sci. Lett. 426, 69–75. doi: 10.1016/j.epsl.2015.05.053
Dansgaard W. (1964). Stable isotopes in precipitation. Tellus 16, 436–468. doi: 10.1111/tus.1964.16.issue-4
Deng C., Xie F., Liu C., Ao H., Pan Y., Zhu R. (2007). Magnetochronology of the Feiliang Paleolithic site in the Nihewan Basin and implications for early human adaptability to high northern latitudes in East Asia. Geophys. Res. Lett. 34, L14301. doi: 10.1029/2007GL030335
Deng T. (2002). The earliest known wooly rhino discovered in the Linxia Basin, Gansu Province, China. Geol. Bull. China 21, 604–608. doi: 10.3969/j.issn.1671-2552.2002.10.002
Deng T. (2006). Neogene rhinoceroses of the Linxia basin (Gansu, China). Cour.-Forschungsinstitut Senckenberg 256, 43–56.
Deng T. (2008). Comparison between woolly rhino forelimbs from Longdan, Northwestern China and Tologoi, Transbaikalian region. Quat. Int. 179, 196–207. doi: 10.1016/j.quaint.2007.09.008
Deng T., Li Q., Tseng Z. J.J., Takeuchi G. T., Wang Y., Xie G. P., et al. (2012). Locomotive implication of a Pliocene three-toed horse skeleton from Tibet and its paleo-altimetry significance. Proc. Natl. Acad. Sci. 109, 7374–7378. doi: 10.1073/pnas.1201052109
Deng T., Lu X., Sun D., Li S. (2023). Rhinocerotoid fossils of the Linxia Basin in northwestern China as late Cenozoic biostratigraphic markers. Palaeogeogr. Palaeoclimatol. Palaeoecol. 614, 111427. doi: 10.1016/j.palaeo.2023.111427
Deng T., Qiu Z. X., Wang B. Y., Wang X. M., Hou S. K. (2013). “Late Cenozoic biostratigraphy of the Linxia Basin, northwestern China,” in Fossil Mammals of Asia: Neogene Biostratigraphy and Chronology. Eds. Wang X. M., Flynn L. J., Fortelius M. (Columbia University Press, New York), 243–273.
Deng T., Wang X., Fortelius M., Li Q., Wang Y., Tseng Z. J., et al. (2011). Out of Tibet: Pliocene woolly rhino suggests high-plateau origin of Ice Age megaherbivores. Science 333, 1285–1288. doi: 10.1126/science.1206594
Dettman D. L., Fang X., Garzione C. N., Li J. (2003). Uplift-driven climate change at 12 Ma: a long δ18O record from the NE margin of the Tibetan plateau. Earth Planet. Sci. Lett. 214, 267–277. doi: 10.1016/S0012-821X(03)00383-2
Dong W., Bai W.-P., Zhang L.-M. (2021). The first description of Rhinocerotidae (Perissodactyla, Mammalia) from Xinyaozi Ravine in Shanxi, North China. Vertebr. Palasiat. 59, 273–294. doi: 10.19615/j.cnki.2096-9899.210715
Dong W., Hou Y., Yang Z., Zhang L., Zhang S., Liu Y. (2014). Late Pleistocene mammalian fauna from Wulanmulan Paleolithic Site, Nei Mongol, China. Quat. Int. 347, 139–147. doi: 10.1016/j.quaint.2014.05.051
Dutton A., Wilkinson B. H., Welker J. M., Bowen G. J., Lohmann K. C. (2005). Spatial distribution and seasonal variation in 18O/16O of modern precipitation and river water across the conterminous USA. Hydrol. Process. 19, 4121–4146. doi: 10.1002/hyp.5876
Farjand A., Zhang Z., Gibbard P. L., Bi S. (2023a). First results of the biostratigraphy and geochronology of the classic Nihewan Fauna, China. Front. Earth Sci. 11, 1276816. doi: 10.3389/feart.2023.1276816
Farjand A., Zhang Z., Kaakinen A., Bi S., Gibbard P. L., Lihua W. (2023b). Rediscovery and stratigraphic calibration of the classic Nihewan Fauna, Hebei Province, China. Quat. Int. 646, 1–10. doi: 10.1016/j.quaint.2022.12.001
Farquhar G. D., Ehleringer J. R., Hubick K. T. (1989). Carbon isotope discrimination and photosynthesis. Annu. Rev. Plant Biol. 40, 503–537. doi: 10.1146/annurev.pp.40.060189.002443
Fortelius M. (1983). The morphology and paleobiological significance of the horns of Coelodonta antiquitatis (Mammalia: Rhinocerotidae). J. Vertebr. Paleontol. 3, 125–135. doi: 10.1080/02724634.1983.10011964
Green D. R., Smith T. M., Green G. M., Bidlack F. B., Tafforeau P., Colman A. S. (2018). Quantitative reconstruction of seasonality from stable isotopes in teeth. Geochim. Cosmochim. Acta 235, 483–504. doi: 10.1016/j.gca.2018.06.013
Hammer O., Harper D. A. T., Ryan P. D. (2001). PAST: paleontological statistics software package for education and data analysis. Electron 4, 9.
Higgins P., MacFadden B. J. (2004). “Amount Effect” recorded in oxygen isotopes of Late Glacial horse (Equus) and bison (Bison) teeth from the Sonoran and Chihuahuan deserts, southwestern United States. Palaeogeogr. Palaeoclimatol. Palaeoecol. 206, 337–353. doi: 10.1016/j.palaeo.2004.01.011
Hodgkins J., Marean C. W., Venter J. A., Richardson L., Roberts P., Zech J., et al. (2020). An isotopic test of the seasonal migration hypothesis for large grazing ungulates inhabiting the Palaeo-Agulhas Plain. Quat. Sci. Rev. 235, 106221. doi: 10.1016/j.quascirev.2020.106221
Hough B. G., Garzione C. N., Wang Z., Lease R. O., Burbank D. W., Yuan D. (2011). Stable isotope evidence for topographic growth and basin segmentation: Implications for the evolution of the NE Tibetan Plateau. Geol. Soc Am. Bull. 123, 168–185. doi: 10.1130/B30090.1
Jin C., Wang Y., Liu J., Ge J., Zhao B., Liu J., et al. (2021). Late Cenozoic mammalian faunal evolution from Jinyuan Cave at Luotuo Hill from Dalian, Northeast China. Quat. Int. 577, 15–28. doi: 10.1016/j.quaint.2021.01.011
Kahlke R.-D. (1999). The history of the origin, evolution and dispersal of the Late Pleistocene Mammuthus-Coelodonta Faunal Complex in Eurasia (large mammals) (Rapid City: Fenske Companies).
Kahlke R.-D. (2014). The origin of Eurasian Mammoth Faunas (Mammuthus–Coelodonta Faunal Complex). Quat. Sci. Rev. 96, 32–49. doi: 10.1016/j.quascirev.2013.01.012
Kahlke R.-D., Lacombat F. (2008). The earliest immigration of woolly rhinoceros (Coelodonta tologoijensis, Rhinocerotidae, Mammalia) into Europe and its adaptive evolution in Palaearctic cold stage mammal faunas. Quat. Sci. Rev. 27, 1951–1961. doi: 10.1016/j.quascirev.2008.07.013
Koch P. L., Fisher D. C., Dettman D. (1989). Oxygen isotope variation in the tusks of extinct proboscideans: A measure of season of death and seasonality. Geology 17, 515–519. doi: 10.1130/0091-7613(1989)017<0515:OIVITT>2.3.CO;2
Kohn M. J. (2010). Carbon isotope compositions of terrestrial C3 plants as indicators of (paleo) ecology and (paleo) climate. Proc. Natl. Acad. Sci. 107, 19691–19695. doi: 10.1073/pnas.1004933107
Kohn M. J., Cerling T. E. (2002). Stable isotope compositions of biological apatite. Rev. Mineral. Geochem. 48, 455–488. doi: 10.2138/rmg.2002.48.12
Kohn M. J., Schoeninger M. J., Valley J. W. (1998). Variability in oxygen isotope compositions of herbivore teeth: reflections of seasonality or developmental physiology? Chem. Geol. 152, 97–112. doi: 10.1016/S0009-2541(98)00099-0
Körner C. h., Farquhar G. D., Wong S. C. (1991). Carbon isotope discrimination by plants follows latitudinal and altitudinal trends. Oecologia 88, 30–40. doi: 10.1007/BF00328400
Krajcarz M., Pacher M., Krajcarz M. T., Laughlan L., Rabeder G., Sabol M., et al. (2016). Isotopic variability of cave bears (δ15N, δ13C) across Europe during MIS 3. Quat. Sci. Rev. 131, 51–72. doi: 10.1016/j.quascirev.2015.10.028
Li Y., Deng T., Hua H., Zhang Y., Wang J. (2020). Isotopic record of palaeodiet of a 7.4 Ma Hipparionine fauna from the central Loess Plateau, northern China: Palaeo-ecological and palaeo-climatic implications. Chem. Geol. 532, 119353. doi: 10.1016/j.chemgeo.2019.119353
Li Z., Dong W. (2007). Mammalian fauna from the Lingjing paleolithic site in Xuchang, Henan Province. Acta Anthropol. Sin. 26, 345–360. doi: 10.3969/j.issn.1000-3193.2007.04.008
Li F., Kuhn S. L., Chen F., Wang Y., Southon J., Peng F., et al. (2018). The easternmost middle paleolithic (Mousterian) from Jinsitai Cave, north China. J. Hum. Evol. 114, 76–84. doi: 10.1016/j.jhevol.2017.10.004
Liu P., Deng C., li S., Cai S., Cheng H., Yuan B., et al. (2012). Magnetostratigraphic dating of the Xiashagou Fauna and implication for sequencing the mammalian faunas in the Nihewan Basin, North China. Palaeogeogr. Palaeoclimatol. Palaeoecol. 315-316, 75–85. doi: 10.1016/j.palaeo.2011.11.011
Ma J., Sun B., Bocherens H., Deng T. (2023). Dietary niche reconstruction of Pliocene and Pleistocene Equidae from the Linxia Basin of northwestern China based on stable isotope analysis. Palaeogeogr. Palaeoclimatol. Palaeoecol. 614, 111416. doi: 10.1016/j.palaeo.2023.111416
Ma J., Wang Y., Baryshnikov G. F., Drucker D. G., McGrath K., Zhang H., et al. (2021). The Mammuthus-Coelodonta Faunal Complex at its southeastern limit: A biogeochemical paleoecology investigation in Northeast Asia. Quat. Int. 591, 93–106. doi: 10.1016/j.quaint.2020.12.024
Ma J., Zhang F., Wang Y., Hu Y. (2017). Tracking the foraging behavior of Mammuthus primigenius from the Late Pleistocene of northeast China, using stable isotope analysis. Quat. Sci. 37, 885–894. doi: 10.11928/j.issn.1001-7410.2017.04.23
Männel T. T., Auerswald K., Schnyder H. (2007). Altitudinal gradients of grassland carbon and nitrogen isotope composition are recorded in the hair of grazers. Glob. Ecol. Biogeogr. 16, 583–592. doi: 10.1111/j.1466-8238.2007.00322.x
Negash E. W., Barr W. A. (2023). Relative abundance of grazing and browsing herbivores is not a direct reflection of vegetation structure: Implications for hominin paleoenvironmental reconstruction. J. Hum. Evol. 177, 103328. doi: 10.1016/j.jhevol.2023.103328
O’Leary M. H. (1981). Carbon isotope fractionation in plants. Phytochemistry 20, 553–567. doi: 10.1016/0031-9422(81)85134-5
Passey B. H., Cerling T. E., Levin N. E. (2007). Temperature dependence of oxygen isotope acid fractionation for modern and fossil tooth enamels. Rapid Commun. Mass Spectrom. 21, 2853–2859. doi: 10.1002/rcm.3149
Pederzani S., Britton K. (2019). Oxygen isotopes in bioarchaeology: Principles and applications, challenges and opportunities. Earth-Sci. Rev. 188, 77–107. doi: 10.1016/j.earscirev.2018.11.005
Pei S., Deng C., de la Torre I., Jia Z., Ma D., Li X., et al. (2019). Magnetostratigraphic and archaeological records at the Early Pleistocene site complex of Madigou (Nihewan Basin): implications for human adaptations in North China. Palaeogeogr. Palaeoclimatol. Palaeoecol. 530, 176–189. doi: 10.1016/j.palaeo.2019.05.014
Peuke A. D., Gessler A., Rennenberg H. (2006). The effect of drought on C and N stable isotopes in different fractions of leaves, stems and roots of sensitive and tolerant beech ecotypes. Plant Cell Environ. 29, 823–835. doi: 10.1111/j.1365-3040.2005.01452.x
Pushkina D., Bocherens H., Ziegler R. (2014). Unexpected palaeoecological features of the Middle and Late Pleistocene large herbivores in southwestern Germany revealed by stable isotopic abundances in tooth enamel. Quat. Int. 339–340, 164–178. doi: 10.1016/j.quaint.2013.12.033
Pushkina D., Juha S., Reinhard Z., Bocherens H. (2020). Stable isotopic and mesowear reconstructions of paleodiet and habitat of the Middle and Late Pleistocene mammals in south-western Germany. Quat. Sci. Rev. 227, 106026. doi: 10.1016/j.quascirev.2019.106026
Qiu Z., Deng T., Wang B. (2004). Early Pleistocene mammalian fauna from Longdan, Dongxiang, Gansu, China (Beijing: Science Press).
Qiu Z., Wang B., Deng T., Ni X., Wang X. (2002). Notes on the mammal fauna from the bottom of loess deposits at Longdan, Dongxiang County, Gansu Province. Quat. Sci. 22, 33–38. doi: 10.3321/j.issn:1001-7410.2002.01.006
Quade J., Cerling T. E., Andrews P., Alpagut B. (1995). Paleodietary reconstruction of Miocene faunas from Paşalar, Turkey using stable carbon and oxygen isotopes of fossil tooth enamel. J. Hum. Evol. 28, 373–384. doi: 10.1006/jhev.1995.1029
Ransom J. I., Kaczensky P. (2016). Wild equids: Ecology, management, and conservation (Baltimore: Johns Hopkins University Press). doi: 10.1353/book.47460
Rey-Iglesia A., Lister A. M., Stuart A. J., Bocherens H., Szpak P., Willerslev E., et al. (2021). Late Pleistocene paleoecology and phylogeography of woolly rhinoceros. Quat. Sci. Rev. 263, 106993. doi: 10.1016/j.quascirev.2021.106993
Rivals F., Álvarez-Lao D. J. (2018). Ungulate dietary traits and plasticity in zones of ecological transition inferred from late Pleistocene assemblages at Jou Puerta and Rexidora in the Cantabrian Region of northern Spain. Palaeogeogr. Palaeoclimatol. Palaeoecol. 499, 123–130. doi: 10.1016/j.palaeo.2018.03.024
Rivals F., Lister A. M. (2016). Dietary flexibility and niche partitioning of large herbivores through the Pleistocene of Britain. Quat. Sci. Rev. 146, 116–133. doi: 10.1016/j.quascirev.2016.06.007
Rivals F., Mihlbachler M. C., Solounias N., Mol D., Semprebon G. M., de Vos J., et al. (2010). Palaeoecology of the Mammoth Steppe fauna from the late Pleistocene of the North Sea and Alaska: Separating species preferences from geographic influence in paleoecological dental wear analysis. Palaeogeogr. Palaeoclimatol. Palaeoecol. 286, 42–54. doi: 10.1016/j.palaeo.2009.12.002
Roberts P., Stewart M., Alagaili A. N., Breeze P., Candy I., Drake N., et al. (2018). Fossil herbivore stable isotopes reveal middle Pleistocene hominin palaeoenvironment in ‘Green Arabia’. Nat. Ecol. Evol. 2, 1871–1878. doi: 10.1038/s41559-018-0698-9
Rozanski K., Araguás-Araguás L., Gonfiantini R. (1993). Isotopic patterns in modern global precipitation. Clim. Change Cont. Isot. Rec. Eds. Swart P. K., Lohmann K. C., McKenzie J. A., Savin S. (American Geophysical Union, Washington), 1–36. doi: 10.1029/gm078p0001
RStudio Team (2016). RStudio: integrated development for R (Version 1.1. 423) (Boston: RStudio Inc).
Schreve D. C. (2001). Differentiation of the British late Middle Pleistocene interglacials: the evidence from mammalian biostratigraphy. Quat. Sci. Rev. 20, 1693–1705. doi: 10.1016/S0277-3791(01)00033-6
Schreve D. (2019). All is flux: the predictive power of fluctuating Quaternary mammalian faunal-climate scenarios. Philos. Trans. R. Soc B Biol. Sci. 374, 20190213. doi: 10.1098/rstb.2019.0213
Schwartz-Narbonne R., Longstaffe F. J., Kardynal K. J., Druckenmiller P., Hobson K. A., Jass C. N., et al. (2019). Reframing the mammoth steppe: Insights from analysis of isotopic niches. Quat. Sci. Rev. 215, 1–21. doi: 10.1016/j.quascirev.2019.04.025
Sharp Z. D., Cerling T. E. (1998). Fossil isotope records of seasonal climate and ecology: Straight from the horse’s mouth. Geology 26, 219–222. doi: 10.1130/0091-7613(1998)026<0219:FIROSC>2.3.CO;2
Smith B. N., Epsten S. (1971). Two categories of 13C/12C ratios for higher plants. Plant Physiol. 47, 380–384. doi: 10.1104/pp.47.3.380
Smith T. M., Martin L. B., Leakey M. G. (2003). Enamel thickness, microstructure and development in Afropithecus turkanensis. J. Hum. Evol. 44, 283–306. doi: 10.1016/S0047-2484(03)00006-X
Sokolowski K. G., Codding B. F., Du A., Faith J. T. (2023). Do grazers equal grasslands? Strengthening paleoenvironmental inferences through analysis of present-day African mammals. Palaeogeogr. Palaeoclimatol. Palaeoecol. 629, 111786. doi: 10.1016/j.palaeo.2023.111786
Sponheimer M., Lee-Thorp J. A. (1999). Oxygen isotopes in enamel carbonate and their ecological significance. J. Archaeol. Sci. 26, 723–728. doi: 10.1006/jasc.1998.0388
Stefaniak K., Kovalchuk O., Ratajczak-Skrzatek U., Kropczyk A., Mackiewicz P., Kłys G., et al. (2023). Chronology and distribution of Central and Eastern European Pleistocene rhinoceros (Perissodactyla, Rhinocerotidae) – A review. Quat. Int. 674–675, 87–108. doi: 10.1016/j.quaint.2023.02.004
Stefaniak K., Stachowicz-Rybka R., Borówka R. K., Hrynowiecka A., Sobczyk A., Moskal-del Hoyo M., et al. (2021). Browsers, grazers or mix-feeders? Study of the diet of extinct Pleistocene Eurasian forest rhinoceros Stephanorhinus kirchbergensis (Jäger 1839) and woolly rhinoceros Coelodonta antiquitatis (Blumenbach 1799). Quat. Int. 605–606, 192–212. doi: 10.1016/j.quaint.2020.08.039
Stuart A. J., Lister A. M. (2012). Extinction chronology of the woolly rhinoceros Coelodonta antiquitatis in the context of late Quaternary megafaunal extinctions in northern Eurasia. Quat. Sci. Rev. 51, 1–17. doi: 10.1016/j.quascirev.2012.06.007
Suga S. (1979). Comparative histology of progressive mineralization pattern of developing incisor enamel of rodents. J. Dent. Res. 58, 1025–1026. doi: 10.1177/002203457905800214011
Tang Z., Liu S., Lin Z., Liu H. (2003). The late Pleistocene fauna from Dabusu of Qian’an in Jilin province of China. Vertebr. Palasiat. 41, 145–153. doi: 10.3969/j.issn.1000-3118.2003.02.005
Tejada-Lara J. V., MacFadden B. J., Bermudez L., Rojas G., Salas-Gismondi R., Flynn J. J. (2018). Body mass predicts isotope enrichment in herbivorous mammals. Proc. R. Soc B Biol. Sci. 285, 20181020. doi: 10.1098/rspb.2018.1020
Tiunov A. V., Kirillova I. V. (2010). Stable isotope (13C/12C and 15N/14N) composition of the woolly rhinoceros Coelodonta antiquitatis horn suggests seasonal changes in the diet. Rapid Commun. Mass Spectrom. 24, 3146–3150. doi: 10.1002/rcm.4755
Tong H. (2004). Paleoenvironmental significance of Coelodonta in different fossil assembledges. Acta Anthr. Sin. 23 (suppl.), 306–314.
Tong H., Li H., Xie J. (2008). Revisions of some taxa of the Salawusu fauna from Sjara-Osso-Gol area, Nei Mongol, China. Quat. Sci. 28, 1106–1113. doi: 10.3321/j.issn:1001-7410.2008.06.016
Tong H., Wang X. (2014). Juvenile skulls and other postcranial bones of Coelodonta nihowanensis from Shanshenmiaozui, Nihewan Basin, China. J. Vertebr. Paleontol. 34, 710–724. doi: 10.1080/02724634.2013.814661
Tong H., Zhang B., Chen X., Wang X., Sun J. (2021). Chronological significance of the mammalian fauna from the Early Pleistocene Shanshenmiaozui site in Nihewan Basin, northern China. Acta Anthr. Sin. 40, 469–489. doi: 10.16359/j.1000-3193/AAS.2021.0026
Troughton J. H., Card K. A. (1975). Temperature effects on the carbon-isotope ratio of C3, C4 and crassulacean-acid-metabolism (CAM) plants. Planta 123, 185–190. doi: 10.1007/BF00383867
Uzunidis A., Antoine P.-O., Brugal J.-P. (2022). A Middle Pleistocene Coelodonta antiquitatis praecursor (Mammalia, Perissodactyla) from Les Rameaux, SW France, and a revised phylogeny of Coelodonta. Quat. Sci. Rev. 288, 107594. doi: 10.1016/j.quascirev.2022.107594
van der Merwe N. J., Medina E. (1991). The canopy effect, carbon isotope ratios and foodwebs in Amazonia. J. Archaeol. Sci. 18, 249–259. doi: 10.1016/0305-4403(91)90064-V
van Geel B., Langeveld B. W., Mol D., van der Knaap P. W. O., van Leeuwen J. F. N. (2019). Pollen and spores from molar folds reflect food choice of late Pleistocene and Early Holocene herbivores in The Netherlands and the adjacent North Sea area. Quat. Sci. Rev. 225, 106030. doi: 10.1016/j.quascirev.2019.106030
Wang B. (2005). Beaver (Rodentia, mammalial) fossils from Longdan, Gansu, China. Vertebr. Palasiat. 43, 237–242. doi: 10.3969/j.issn.1000-3118.2005.03.008
Wang Y., Deng T. (2005). A 25 m.y. isotopic record of paleodiet and environmental change from fossil mammals and paleosols from the NE margin of the Tibetan Plateau. Earth Planet. Sci. Lett. 236, 322–338. doi: 10.1016/j.epsl.2005.05.006
Wang H., Deng C., Zhu R., Wei Q., Hou Y., Boëda E. (2005). Magnetostratigraphic dating of the Donggutuo and Maliang Paleolithic sites in the Nihewan Basin, North China. Quat. Res. 64, 1–11. doi: 10.1016/j.yqres.2005.04.001
Wang Y., Kromhout E., Zhang C., Xu Y., Parker W., Deng T., et al. (2008a). Stable isotopic variations in modern herbivore tooth enamel, plants and water on the Tibetan Plateau: Implications for paleoclimate and paleoelevation reconstructions. Palaeogeogr. Palaeoclimatol. Palaeoecol. 260, 359–374. doi: 10.1016/j.palaeo.2007.11.012
Wang H., Li Z., Tong H., van Kolfschoten T. (2021). Hominin paleoenvironment in East Asia: The Middle Paleolithic Xuchang-Lingjing (China) mammalian evidence. Quat. Int. 633, 118–133. doi: 10.1016/j.quaint.2021.11.024
Wang X., Li Q., Xie G., Saylor J. E., Tseng Z. J., Takeuchi G. T., et al. (2013). Mio-Pleistocene Zanda Basin biostratigraphy and geochronology, pre-Ice Age fauna, and mammalian evolution in western Himalaya. Palaeogeogr. Palaeoclimatol. Palaeoecol. 374, 81–95. doi: 10.1016/j.palaeo.2013.01.007
Wang Y., Wang X., Xu Y., Zhang C., Li Q., Tseng Z. J., et al. (2008b). Stable isotopes in fossil mammals, fish and shells from Kunlun Pass Basin, Tibetan Plateau: Paleo-climatic and paleo-elevation implications. Earth Planet. Sci. Lett. 270, 73–85. doi: 10.1016/j.epsl.2008.03.006
Wang Y., Xu Y., Khawaja S., Passey B. H., Zhang C., Wang X., et al. (2013). Diet and environment of a mid-Pliocene fauna from southwestern Himalaya: Paleo-elevation implications. Earth Planet. Sci. Lett. 376, 43–53. doi: 10.1016/j.epsl.2013.06.014
Wickham H. (2011). ggplot2: Elegant Graphics for Data Analysis. Wiley Interdisc. Rev. Comput. Stat. 3, 180–185. doi: 10.1002/wics.147
Xu Z., Pei S., Hu Y., de la Torre I., Ma D. (2021). Stable isotope analysis of mammalian enamel from the Early Pleistocene site of Madigou, Nihewan Basin: Implications for reconstructing hominin paleoenvironmental adaptations in North China. Front. Earth Sci. 9, 789781. doi: 10.3389/feart.2021.789781
Xu Z., Pei S., Hu Y., de la Torre I., Ma D., Ye Z., et al. (2023). Ecological shifts and hominin adaptations during the Mid-Pleistocene Climate Transition in Northeast Asia as evidenced by isotopic analysis (δ13C, δ18O) of mammalian enamel from Early Paleolithic sites in the Nihewan Basin, China. Quat. Sci. Rev. 308, 108072. doi: 10.1016/j.quascirev.2023.108072
Xue X., Zhang Y., Yue L. (2006). Paleoenvironments indicated by the fossil mammalian assemblages from red clay-loess sequence in the Chinese Loess Plateau since 8.0 Ma B.P. Sci. China Ser. D 49, 518–530. doi: 10.1007/s11430-006-0518-y
Yang S., Ding Z., Wang X., Tang Z., Gu Z. (2012). Negative δ18O–δ13C relationship of pedogenic carbonate from northern China indicates a strong response of C3/C4 biomass to the seasonality of Asian monsoon precipitation. Palaeogeogr. Palaeoclimatol. Palaeoecol. 317–318, 32–40. doi: 10.1016/j.palaeo.2011.12.007
Zan J., Fang X., Zhang W., Yan M., Zhang T. (2016). Palaeoenvironmental and chronological constraints on the Early Pleistocene mammal fauna from loess deposits in the Linxia Basin, NE Tibetan Plateau. Quat. Sci. Rev. 148, 234–242. doi: 10.1016/j.quascirev.2016.07.026
Zhang C., Wang Y., Li Q., Wang X., Deng T., Tseng Z. J., et al. (2012). Diets and environments of late Cenozoic mammals in the Qaidam Basin, Tibetan Plateau: Evidence from stable isotopes. Earth Planet. Sci. Lett. 333–334, 70–82. doi: 10.1016/j.epsl.2012.04.013
Keywords: Longdan fauna, Coelodonta nihowanensis, stable isotopes, paleoecology, paleoenvironment, Early Pleistocene, woolly rhinoceros
Citation: Ma J, Wang S and Deng T (2024) When the woolly rhinoceroses roamed East Asia: a review of isotopic paleoecology of the genus Coelodonta from the Tibetan Plateau to northern Eurasia. Front. Ecol. Evol. 12:1377000. doi: 10.3389/fevo.2024.1377000
Received: 16 February 2024; Accepted: 21 March 2024;
Published: 11 April 2024.
Edited by:
Florent Rivals, Institut Català de Paleoecologia Humana i Evolució Social (IPHES), SpainReviewed by:
Maciej Tomasz Krajcarz, Polish Academy of Sciences, PolandNaoto Handa, Osaka University, Japan
Copyright © 2024 Ma, Wang and Deng. This is an open-access article distributed under the terms of the Creative Commons Attribution License (CC BY). The use, distribution or reproduction in other forums is permitted, provided the original author(s) and the copyright owner(s) are credited and that the original publication in this journal is cited, in accordance with accepted academic practice. No use, distribution or reproduction is permitted which does not comply with these terms.
*Correspondence: Tao Deng, dengtao@ivpp.ac.cn