Introduction
Glaucoma and uveitis are non-vascular ocular diseases which are among the leading causes of blindness and visual loss (Ref. 1). These chronic conditions pose a significant social and economic burden resulting from their prevalence and impact on patients' mental health, independence, work productivity and career, in addition to treatment costs. Hence, reduced quality of life is often experienced by affected patients, which are usually in the working age group (Refs Reference Aboobakar and Wiggs2, Reference Dhawan3, Reference Patel4, Reference Shome5). Both conditions encompass a complex, multifactorial group of entities, with the clinical presentation of progressive degeneration of retinal ganglion cells (RGCs) or intraocular inflammation being the basis of diagnosis (Refs Reference Aboobakar and Wiggs2, Reference Shome5). The exact pathogenesis of both diseases is not entirely understood, but it is known that genetic, environmental and immunological factors play important roles (Refs Reference Huang and Brown6, Reference Sim7, Reference Wiggs8).
Achieving good outcomes in patients with glaucoma and the non-infectious form of uveitis may be challenging for clinicians because of the complex nature of these diseases and delayed definitive diagnosis (Refs Reference Martucci, Nucci and Pinazo-Duran9, Reference Singh10). Current management of glaucoma includes surgical and pharmacological interventions, both aiming at reducing intraocular pressure (IOP), the primary and only modifiable risk factor associated with the disease, a strategy that has not changed in 100 years (Ref. Reference Aboobakar and Wiggs2). Thus, IOP lowering to a target range by instilling eye drops from various pharmacological classes or performing laser therapy on the trabecular meshwork (TM) is the initial approach (Ref. Reference Sim7). However, IOP control alone is insufficient to halt glaucoma progression in some patients, which, along with high levels of non-compliance, can lead to therapeutic failure and potentially vision loss (Refs Reference Adams11, Reference Shalaby12). Therefore, novel IOP-lowering approaches less dependent upon patient compliance and therapeutic options targeting non-IOP-related risk factors are needed to improve management of glaucoma (Refs Reference Martucci, Nucci and Pinazo-Duran9, Reference Adams11). Similarly, the standard of care for uveitis has remained the same since the 1950s and relies on immunosuppression by corticosteroids (CS) administered by multiple routes (Ref. Reference Shome5). Unfortunately, CS are sometimes ineffective and often associated with severe local and systemic side effects, especially in long-term treatment, and this limits their clinical utility. These limitations restrict the use of CS and highlight the need for alternative therapies with better safety profiles and proven efficacy (Refs Reference Castro13, Reference Rosenbaum14).
Extensive research has been conducted to improve understanding of underlying mechanisms driving these chronic, difficult to treat ophthalmic conditions, and several novel therapeutic approaches have been explored. These strategies include novel agents, nanocarriers, extended-release devices and gene- and cell-based therapies, each with unique advantages and relevance to the field, which have been reviewed elsewhere (Refs Reference Patel4, Reference Singh10, Reference Adams11, Reference Shalaby12, Reference Bordet15, Reference Egwuagu, Alhakeem and Mbanefo16, Reference Ghanchi17, Reference Gilger and Hirsch18, Reference Khatib and Martin19, Reference Komaromy, Koehl and Park20, Reference Nuzzi and Tridico21, Reference Rolle, Ponzetto and Malinverni22, Reference Shen, Wang and Yao23, Reference Skopinski24, Reference Thomas25).
Gene therapy has emerged as a promising approach for treating various acquired ocular disorders in addition to its established role in genetic ophthalmic disease. Adeno-associated virus (AAV) is the leading vector in ocular gene delivery because of its relatively low immunogenicity, ability to transduce a wide range of cells and versatility (Ref. Reference Whitehead26). Although other approaches can undoubtedly bring benefits to glaucoma and uveitis patients, AAV stands out as a promising technology given the possibility of optimisation of the delivery and expression methods, including regulation, targeting, specificity and multi-acting, in addition to a scalable manufacturing process (Refs Reference Castro, Steel and Layton27, Reference Dobrowsky28). These distinctive features underscore the potential of AAV to address challenges associated with the management of prevalent, chronic, multifactorial and complex disorders (Ref. Reference Castro, Steel and Layton27).
Recent findings from clinical trials involving AAV-based gene therapies for acquired vascular diseases offer promising insights into the potential extension of this technology to non-vascular ophthalmic conditions, including uveitis and glaucoma. In fact, preclinical studies have reported positive outcomes with constructs targeting multiple mechanisms, including neuroprotection, immunomodulation, oxidative stress or aqueous humour outflow in glaucoma, and the complement system, the inflammasome and immune tolerance in uveitis. Optimised designs, such as cell-specific promoters, multigenic cassettes and inducible systems, have been explored, in addition to gene editing, gene silencing and capsid engineering technology (Refs Reference Jiang29, Reference Lani-Louzada30, Reference Luo31, Reference Osborne32, Reference Phatak, Stankowska and Krishnamoorthy33). Gene therapies encoding anti-inflammatory cytokines or peptides have also been proposed for both diseases (Refs Reference Crabtree34, Reference Crabtree35, Reference Li36, Reference Lo37, Reference Ridley38).
Unfortunately, clinical translation has yet to be achieved despite the successful results observed in animal models. Accomplishing this is made more challenging because, unlike incurable genetic eye diseases, any therapy for these conditions must be evaluated against current standard-of-care treatments. Hence, their acceptance and broad adoption will only occur if compelling evidence of their advantages over existing solutions is seen (Ref. Reference Tan39).
Therefore, it is important to discuss therapeutic and delivery strategies reported in preclinical studies for glaucoma and uveitis, with their respective benefits, limitations and experimental strategy to pave the way to the development of therapies with improved prospects of advancing to clinical studies. Hence, this review provides an overview of AAV-based strategies which have reached preclinical animal or human testing in these multifactorial chronic diseases. We explore therapeutic and delivery approaches such as vector modification, expression cassette optimisation and gene of interest application. We delve into the potential limitations of current therapeutic approaches and discuss the ongoing challenges associated with gene therapy for these chronic, partially treatable ocular diseases.
Glaucoma
Background and prevalence
Glaucoma is a complex group of optic neuropathies characterised by progressive degeneration of RGCs and nerve fibre layers and damage to the optic nerve, resulting in irreversible vision loss (Refs Reference Aboobakar and Wiggs2, Reference Nuzzi and Tridico21). Glaucoma was the leading cause of irreversible blindness globally in 2020 and is estimated to be affecting 111.8 million people in 2040 (Refs 1, Reference Dhurandhar40). The prevalence of glaucoma varies by geographic area and seems to rise as the population ages, posing a significant financial and clinical burden, which increases with the disease severity (Refs Reference Shih41, Reference Sun42). Different genetic and environmental risk factors have been described, including elevated IOP, older age, African background, family history, high myopia, vascular disease and obesity (Refs Reference Nuzzi and Tridico21, Reference Shen, Wang and Yao23, Reference Jonas43, Reference Wang44, Reference Wang and Wiggs45). Glaucoma can be classified as primary if idiopathic (unknown cause) or secondary when an underlying condition or event is identified (Ref. Reference Sim7), or according to the anatomy of the iridocorneal angle as open-angle or angle-closure. Secondary types of glaucoma include pigmentary, congenital, uveitic, traumatic, neovascular and exfoliative (Refs Reference Shen, Wang and Yao23, Reference Becker, Fakhiri and Grimm46, Reference Munoz-Negrete47). Primary open angle is the most common type of glaucoma. Chronic glaucoma often progresses asymptomatic until substantial RGC degeneration has occurred at advanced stages (Ref. Reference Weinreb, Aung and Medeiros48). The visual impairment at this stage can manifest as blurriness, blind spots, impaired contrast and colour perception and loss of peripheral visual acuity. These clinical symptoms directly affect patients' quality of life by limiting their mobility and ability to perform daily activities, such as driving and reading. Early detection of glaucoma is essential to save patients' sight and improve prognosis (Refs Reference Weinreb, Aung and Medeiros48, Reference Sharif49).
Pathogenesis and management
The disease pathogenesis is complex and has not been completely elucidated. However, elevated IOP is related to the loss of RGCs and their axons, along with excavation of the optic nerve head, which is present in all forms of glaucoma (Refs Reference Sim7, Reference Shen, Wang and Yao23). Elevation of the IOP, in turn, happens as a result of an imbalance between aqueous humour secretion and drainage, with the latter occurring either through the TM and Schlemm's canal or via the uveoscleral outflow pathway (Refs Reference Jonas43, Reference Weinreb, Aung and Medeiros48). Yet, as people with IOP at a normal range can also develop the disease (‘normal tension glaucoma’), other mechanisms are implicated in the disease pathogenesis (Ref. Reference Weinreb, Aung and Medeiros48). Therefore, the complex mechanism driving neurodegeneration in glaucoma seems to involve mechanical, vascular, genetic and immunological factors (Ref. Reference Sim7). Furthermore, oxidative stress, ischaemia, deficiency of neurotrophic factors, glial activation and excitotoxicity have been suggested as important processes in glaucoma development and represent important targets for neuroprotective therapies (Refs Reference Skopinski24, Reference Osborne50).
Current management of glaucoma aims to lower the IOP at a target level and thereby slow degeneration, and this is achieved with topical hypotensive agents, laser therapy and surgery (Ref. Reference Jonas43). However, low adherence to a chronic treatment involving multiple applications, encouraged by the absence of symptoms at even moderately late stages of the disease, and aggravated by incorrect administration of eye drops, contributes to treatment failure (Refs Reference Adams11, Reference Nuzzi and Tridico21). In some patients, the individualised target IOP is not achieved with therapeutic interventions with acceptable side effects, resulting in continued disease progression and worsening visual impairment, thereby impacting the quality of life. In these cases, laser trabeculoplasty or incisional surgical procedures may be indicated (Refs Reference Weinreb, Aung and Medeiros48, Reference Boccaccini51). Also, since IOP control alone is not enough to prevent glaucoma progression in some patients, investigation of additional therapies is needed (Refs Reference Shalaby12, Reference Skopinski24). In this context, gene therapies may benefit glaucoma patients by either targeting genes associated with the condition or inducing the expression of factors which influence the pathogenesis (Refs Reference Shalaby12, Reference Komaromy, Koehl and Park20). Indeed, as mentioned, AAV vectors have been widely employed to deliver genes encoding molecules with neuroprotective, antioxidant, antiapoptotic or anti-inflammatory effects, as well as those affecting the aqueous humour outflow (Table 1).
Table 1. Preclinical studies using AAV-mediated gene therapies in models of glaucoma

AAV, adeno-associated virus; vg, vector genome; ABCA1, ATP-binding cassette transporter A1; Bcl-2, B cell leukaemia/lymphoma 2; Bcl-xL, B cell lymphoma extra-large; BDNF, brain-derived neurotrophic factor; Brn3b, brain-specific homoeobox/POU domain protein 3b; C3, C3 exoenzyme transferase; CAG, cytomegalovirus enhancer – chicken beta-actin hybrid promoter; CMV, cytomegalovirus; CRISPR, clustered regularly interspaced short palindromic repeats; hSyn, human synapsin 1 gene promoter; IOP, intraocular pressure; MAX, MYC-associated protein X; MCP-1, monocyte chemoattractant protein-1; MMP-3, matrix metalloproteinase-3; Nrf2, nuclear factor erythroid 2-related factor 2; Pgk, phosphoglycerokinase; scAAV2, self-complementary AAV2; SOD2, superoxide dismutase 2; TrkB, tropomyosin-related receptor kinase-B.
Gene therapy approaches
AAV-mediated gene therapies targeting neuroprotection
Gene therapies providing neuroprotection for RGCs can either target natural survival pathways or prevent RGCs from progressing to cell death (Ref. Reference Lani-Louzada30). The first approach can be accomplished by overexpressing neurotrophic factors, such as the brain-derived neurotrophic factor (BDNF), which has shown therapeutic potential in multiple animal models of glaucoma (Refs Reference Osborne32, Reference Igarashi52, Reference Martin53, Reference Ren54, Reference Shiozawa55, Reference Wojcik-Gryciuk56). Although BDNF overexpression has indeed led to neuroprotection in these studies, its long-term effect, desired in therapies for chronic diseases, is hindered partly because of the downregulation of its receptor, which seems to occur in response to the overexpression (Ref. Reference Osborne32). Thus, to overcome it, Osborne et al. developed a dual-acting AAV2 system co-expressing BDNF and its receptor, the tropomyosin-related receptor kinase-B (TrkB) (Ref. Reference Osborne32). The therapy was injected intravitreally in mice and rats before optic nerve crush and laser-induced IOP elevation models, respectively. Expression of both proteins for up to 6 months was reported, along with RGC survival and functional improvements, with better neuroprotection seen with the dual system compared with each transgene alone. The expression of proteins involved in BDNF/TrkB signalling-mediated pathways was also demonstrated. Yet, more studies should be conducted to ensure the safety of this therapy since elevated expression of BDNF/TrkB has been associated with increased glutamate excitotoxicity, which may impair neuronal homoeostasis (Ref. Reference Mallone57).
Conversely, Wojcik-Gryciuk et al. argue that there is a correlation between BDNF overexpression and long-term TrkB downregulation (Ref. Reference Wojcik-Gryciuk56). The authors observed that moderate overexpression of BDNF mediated by AAV2 vectors did not result in downregulation of TrkB and led to long-term neuroprotection (6 weeks after disease induction). The outcome was achieved with intravitreal delivery of the therapy given before induction of the trabecular occlusion model of glaucoma. The authors proposed control of BDNF levels as the critical factor for long-term responsiveness of RGCs and maintenance of TrkB levels. However, the dose utilised to achieve this moderate expression was two times higher than the high dose used by Osborne et al. (Ref. Reference Osborne32), which led to improved neuroprotection when BDNF was co-expressed with the receptor, although different animal models and time-points were assessed. Still, knowing that BDNF can also activate signalling pathways promoting cell death and that both BDNF and TrkB are expressed in malignant gliomas, a controlled expression of these proteins may be the best strategy to enable their safe practical application in glaucoma gene therapies and enable modulation of the expression levels (Ref. Reference Lawn58).
An alternative approach targeting neuroprotection was investigated by Stankowska et al. (Ref. Reference Stankowska59) and Phatak et al. (Ref. Reference Phatak, Stankowska and Krishnamoorthy33), who employed AAV2 vectors packaging the brain-specific homoeobox/POU domain protein 3b (Brn3b), a transcription factor that controls RGC development [(Refs Reference Phatak, Stankowska and Krishnamoorthy33, Reference Stankowska59). Stankowska et al. (Ref. Reference Stankowska59) proposed a neuron-specific strategy by employing the human synapsin 1 promoter (hSyn). The study reported neuroprotective effects in axons of the optic nerve and RGCs, accompanied by partially restored visual function in animals subjected to Morrison's model of glaucoma. The same animal model was used in Phatak et al. to assess the efficacy of a constitutively expressed construct which was intravitreally injected after disease induction (Ref. Reference Phatak, Stankowska and Krishnamoorthy33). Protection of RGCs and their axons was found in rats treated with AAV2-Brn3b, and it was attributed to the upregulation of B cell leukaemia/lymphoma 2 (Bcl-2) (Ref. Reference Phatak, Stankowska and Krishnamoorthy33). The B cell lymphoma extra-large (Bcl-xL) antiapoptotic protein was also upregulated in Brn3b-treated animals, although not statistically different from green fluorescent protein (GFP) controls. Bcl-xL is a member of the Bcl-2 family predominantly expressed in retinas and plays an essential role in RGC survival (Ref. Reference Phatak, Stankowska and Krishnamoorthy33). Donahue et al. reported the efficacy of Bcl-xL overexpression through AAV2 vectors in two models of glaucoma, the optic nerve crush and DBA/2J mice (Ref. Reference Donahue60). The gene therapy contained a neuron-specific promoter, the phosphoglycerokinase, for RGC-targeted expression of the antiapoptotic protein. The formulation was injected intravitreally just before DBA/2J mice exhibited elevated IOP. Although this therapy did not inhibit IOP increase, Bcl-xL protected RGCs from degeneration in both models. Despite the positive outcome, the authors drew attention to potential safety concerns in long-term overexpression of antiapoptotic proteins, owing to their possible effect on oncogenesis, as discussed elsewhere (Ref. Reference Lani-Louzada30). Therefore, vector optimisation incorporating elements for a targeted and regulated expression may be the most suitable approach and increase the chance of clinical translation of gene therapies encoding antiapoptotic proteins and other therapeutic molecules whose continuous or widespread overexpression might be harmful.
Lani-Louzada et al. sought to avoid potential teratogenic side effects associated with antiapoptotic proteins and neurotrophic factors by utilising a tumour suppressor gene, the MYC-associated protein X (MAX) (Ref. Reference Lani-Louzada30). MAX was packaged into AAV2 vectors and intravitreally delivered to rats before optic nerve crush or the limbal plexus cautery models of glaucoma (Ref. Reference Lani-Louzada30). The therapy did not affect IOP dynamics and prevented RGC degeneration in both models, with no adverse events reported. However, further studies should assess systemic transgene expression and address potential side effects, given the ubiquitous nature of MAX and the promoter used, in addition to the likelihood of systemic exposure after intravitreal injection and the broad tropism of AAV2 (Ref. Reference Srivastava61). Nevertheless, positive findings reported with MAX encourage the investigation of similar genes in neurodegenerative diseases and may expand the range of candidates for glaucoma gene therapy.
Attempts have also been made to overcome the theoretical detrimental effects of unspecific expression of neurotrophic factors by using physiologically induced promoters. Fujita et al. aimed to direct the expression to cells at risk of degeneration by utilising the monocyte chemoattractant protein-1 (MCP-1), an early-stress inducible promoter shown to be upregulated preceding RGC death (Ref. Reference Fujita62). AAV2 vectors were used to deliver an MCP-1 promoter driving the expression of either nuclear factor erythroid 2-related factor 2 (Nrf2) a regulator of multiple antioxidant genes or BDNF (Ref. Reference Fujita62). Intravitreal treatment with one of the two therapies in mice subjected to the optic nerve crush model resulted in decreased cell death and higher expression of RGC markers, with Nrf2 leading to better RGC neuroprotection. Comparative analysis between constitutive and MCP-1-controlled expression of Nrf2 revealed similar therapeutic effects in terms of RGC protection and contrast sensitivity, although both strategies could not improve visual acuity (Ref. Reference Fujita62). Since Nrf2 seems to promote RGC degeneration when constitutively expressed, Fujita et al. (Ref. Reference Fujita62) seem to have enabled safe application of Nrf2 in an injury model of glaucoma by timely and spatially modulating its expression with a stress-inducible promoter.
AAV-mediated gene therapies targeting oxidative stress and inflammation
Alternative gene therapies for glaucoma seek to counteract the oxidative stress and inflammation involved in the disease pathogenesis. For instance, Jiang et al. used AAV2 vectors encoding superoxide dismutase 2 (SOD2), an antioxidant enzyme, in rats subjected to a chronic glaucoma model induced by laser burns at the TM and episcleral veins (Ref. Reference Jiang29). The therapy was delivered intravitreally before disease induction, and expression of SOD2 was detected 4 weeks after laser burns. Although RGC survival and preservation of mitochondrial function were reported, these effects did not endure, and complete elimination of the oxidative stress was not achieved (Ref. Reference Jiang29). Still, pretreatment with AAV2-SOD2 increased the activity/expression of antioxidant enzymes and decreased retinal malondialdehide content, a marker of oxidative stress (Ref. Reference Jiang29). Luo et al. used AAV8 vectors to express a fragment of ATP-binding cassette transporter A1 (ABCA1), thought to regulate the nuclear translocation of the anti-inflammatory molecule annexin A1 (ANXA1), a process associated with induction of apoptosis in neuronal cells (Ref. Reference Luo31). Intravitreal treatment in mice later subjected to the ischaemia/reperfusion model led to reduced ANXA1 nuclear translocation and prevented RGC degeneration. Interestingly, this was achieved with the expression of a fragment containing only 441 amino acids, whereas the entire length of this protein is 2261 amino acids long (Ref. Reference Luo31). In fact, the authors tested two different fragments of ABCA1 and only the section containing the amino acids 903–1344 demonstrated therapeutic effects (Ref. Reference Luo31). Nevertheless, this therapy was ineffective when treating the animals after disease induction, suggesting that existing cell damage could affect its efficacy, a limitation commonly observed in gene therapies for glaucoma. Still, several other targets implicated in decreasing or preventing neuroinflammation in glaucoma have been identified and may be explored in future preclinical investigations. Despite limitations, SOD2 and ABCA1 exhibited encouraging results regarding their respective therapeutic properties. Therefore, both therapies might benefit from vectors with broader tropism to achieve a long-lasting effect independent of cells highly affected during the disease course, as reported with certain engineered vectors. Notably, the AAV2.7m8 has shown effective retinal transduction following both intravitreal and subretinal delivery in degenerated mice retina, as well as in healthy explants from human donors and primates (Ref. Reference Hickey63).
AAV-mediated gene therapies targeting the aqueous humour outflow
Given the significance of aqueous humour outflow regulation on glaucoma pathogenesis, additional strategies have explored the expression of molecules involved in this process as potential gene therapies. Tan et al. assessed the efficacy of overexpression of C3 exoenzyme transferase (C3), a molecule which disrupts the actin cytoskeleton and cellular adhesion in TM cells by inactivating Rho, whose signalling pathway is crucial in IOP regulation (Refs Reference Tan64, Reference Tan65). Using a self-complementary AAV2 (scAAV2), intracameral C3 expression lowered IOP in healthy monkeys and mice despite some adverse reactions (Refs Reference Tan64, Reference Wang66). scAAV is a modified vector with a double-stranded genome and half-load capacity often used when targeting the anterior segment because of the reported inability of TM cells to convert AAV single-stranded DNA into a double-strand (Ref. Reference Lee67).
This preliminary test assessed the intracameral administration of scAAV2-C3, but the same authors utilised the intravitreal route to investigate this therapy in rats subjected to the ischaemia/reperfusion injury model. The treatment protected retinal neuronal cells from damage and alleviated retinal thickness reduction (Ref. Reference Tan65). The therapy also led to decreased expression of apoptotic markers and increased RGC survival. The outcomes were attributed to the effect of C3 on Rho GTPases involved in cell proliferation and apoptosis (Ref. Reference Tan65). The multiple effects of C3 targeting different processes involved in the pathogenesis of glaucoma make it an attractive candidate for glaucoma therapy. However, questions remain regarding the safety of intravitreal scAAV2-C3 and whether transduction of TM cells and lower IOP are achieved by this route. Although intracameral administration delivers the therapeutic agent directly into the anterior chamber, drugs injected intravitreally primarily target the retina but can be eliminated through the anterior route by diffusion and using the aqueous humour turnover (Ref. Reference Varela-Fernandez68). Furthermore, scAAV2 seems to be a suitable vector for intracameral delivery, whereas for intravitreal administration, the single-stranded vector exhibits higher transduction efficiency (Ref. Reference Tan65).
Another effort to optimise cellular targeting was made by Wu et al., who chose AAVshH10 for a glaucoma gene therapy after an initial study showed its more efficient transduction in ciliary body non-pigmented epithelium with intravitreal injection when compared with four other serotypes (Ref. Reference Wu69). Additionally, the study leveraged gene editing technology to reduce aqueous humour production by depleting aquaporins. A small Cas9 variant, the Staphylococcus aureus-derived CRISPR-Cas9 system was packaged into AAVshH10 vectors along with one of two short guide RNAs, both selected for their efficacy and exon location. The authors first showed that treatment with a mix of AAV formulations delivering each of the two short guide RNAs was more beneficial than each gene therapy alone. Hence, an intravitreal treatment containing 1:1 mix of the two therapies was given to mice 1 week after CS- and microbead-induced models of ocular hypertension. Although Aqp1 is present in various ocular tissues and a ubiquitous promoter was employed, selected Aqp1 disruption in the ciliary body was achieved with this combined therapy, with a reduction in IOP and protection of RGC reported in both disease models. However, decreasing aqueous humour production is a mechanic approach only, which does not affect all other pathophysiological processes that lead to glaucoma. Hence, the therapy might not have a long-term therapeutic effect or slow disease progression (Refs Reference Komaromy, Koehl and Park20, Reference Killer and Pircher70). Nevertheless, combining small gene editing systems with engineered capsids brings a novel perspective on targeted gene therapy for glaucoma. It is noteworthy, however, that the application of gene editing therapies is still limited because of safety concerns regarding off-target effects and oncogenesis (Ref. Reference He71). Still, gene editing research is quickly advancing with more clinical trials underway, which might address these limitations and ease the clinical translation of this technology.
The aqueous humour outflow and IOP homoeostasis are also affected by the activity of matrix metalloproteinases (MMPs), a family of extracellular matrix (ECM) modifying proteases expressed in the eye and most tissues (Ref. Reference O'Callaghan72). O'Callaghan et al. explored the role of the MMP type 3 (MMP-3), a small protease that degrades the ECM, in glaucoma and developed a gene therapy consisting of AAV2/9 delivering MMP-3, under the control of the tetracycline-inducible promoter (Ref. Reference O'Callaghan72). The tetracycline-inducible system in the configuration used in the study reversibly activates gene expression in the presence of doxycycline. The therapy was assessed in two murine models of glaucoma, dexamethasone-induced ocular hypertension and the transgenic mouse with myocilin Y437H mutation (Tg-MYOCY437H). Intracameral injection of the therapy was given before dexamethasone administration for the first model or in 4-month-old Tg-MYOCY437H mice for the second model. Treatment with doxycycline eye drops twice daily for 2 weeks resulted in decreased IOP and signs of ECM degradation in both disease models, with no alterations in control animals. Increased outflow facility was reported in glaucomatous and control eyes, although the latter showed a higher raise. Similarly, increase in outflow facility by MMP-3 delivered by the same vector and injection route was reported in healthy non-human primates (NHPs) and human donor eyes, although with a constitutive expression system (Ref. Reference O'Callaghan72). Interestingly, the authors also developed a similar gene therapy with a codon-optimised sequence of MMP-3, which increased the transgene expression in vitro (human cells) and in vivo (mice and NHPs) compared with the native form. However, only the native form was assessed in the disease models. Therefore, the approaches described by O'Callaghan et al. (Ref. Reference O'Callaghan72) hold great promise for glaucoma therapy, considering that individualised doxycycline treatments may improve disease control in patients with different clinical settings, although it would create an added compliance burden. Furthermore, the inducible strategy is relevant given that MMPs and their inhibitors are highly expressed in other organs, and off-target effects could lead to proteolysis (Ref. Reference O'Callaghan73). In addition, the increased expression levels achieved with the codon-optimised sequence may contribute to reducing vectors' dosage and improve the safety of the gene therapy should further investigation prove its efficacy in disease models.
Current progress and future directions
Collectively, these diverse approaches (Fig. 1; Table 2) underscore the difficulty of planning and designing gene therapies for a multifactorial chronic disease with slow progression, such as glaucoma. In addition, the virtual impossibility of mirroring a complex pathogenesis and disease course in experimental models and the need for long-duration trials to accurately assess drug candidates make the development process very challenging. Nevertheless, one potential strategy to address some of these obstacles is employing gene therapies that deliver dual-acting cassettes, which can simultaneously affect multiple processes and pathways implicated in the disease pathogenesis. Additionally, engineered vectors, cell-specific promoters and inducible systems may help mitigate safety issues, given that a significant number of studies reported concerns with the overexpression of various genes of interest, emphasising the importance of carefully managing dosage, off-target effects and expression levels. Furthermore, with extensive ongoing research, an in-depth understanding of disease mechanisms and prospective targets is on track. Advances in ophthalmic imaging techniques have also allowed better monitoring of patients and may contribute to improved selection of patients and endpoints in clinical trials.

Figure 1. Overview of delivered genes and pathophysiological targets of recent preclinical studies with AAV-mediated gene therapies for the treatment of glaucoma. AAV, adeno-associated virus; ABCA1, ATP-binding cassette transporter A1; Bcl-xL, B cell lymphoma extra-large; BDNF, brain-derived neurotrophic factor; Brn3b, brain-specific homoeobox/POU domain protein 3b; C3, C3 exoenzyme transferase; CRISPR, clustered regularly interspaced short palindromic repeats; MAX, MYC-associated protein X; MMP-3, matrix metalloproteinase-3; Nrf2, nuclear factor erythroid 2-related factor 2; SOD2, superoxide dismutase 2; TrkB, tropomyosin-related receptor kinase-B.
Table 2. Strategies and main outcomes of preclinical studies using AAV-mediated gene therapies in models of glaucoma

AAV, adeno-associated virus; ABCA1, ATP-binding cassette transporter A1; Bcl-2, B cell leukaemia/lymphoma 2; Bcl-xL, B cell lymphoma extra-large; BDNF, brain-derived neurotrophic factor; IOP, intraocular pressure; MAX, MYC-associated protein X; RGC, retinal ganglion cells; SOD2, superoxide dismutase 2; TrkB, tropomyosin-related receptor kinase-B; AKT, protein kinase B.
Uveitis
Background and prevalence
An eye is an immune-privileged organ because of multiple mechanisms, including molecular and anatomical features regulating intraocular innate and adaptive responses (Ref. Reference Keino, Horie and Sugita74). Yet, this highly nuanced defence can fail or become overloaded, leaving eye tissues susceptible to inflammation and infection (Refs Reference Merida75, Reference Molzer76). Uveitis refers to inflammation of the uveal tract, composed of the iris, ciliary body and choroid, and can also affect adjacent structures. Uveitis is a heterogeneous group of sight-threatening conditions, accounting for 10–20% of preventable blindness cases in developed countries and 25% in the developing world (Refs Reference Patel4, Reference Hou77). It can be an isolated disease or an ocular manifestation of systemic syndromes (Ref. Reference Perez and Caspi78). Severe disease, inadequate disease management or lack of treatment can lead to severe vision loss and blindness because of complications including cataracts, glaucoma, vitreous debris, retinopathy and, most commonly, macular oedema (Refs Reference Dick79, Reference Prieto-Del-Cura and Gonzalez-Guijarro80). The latter is the main condition related to vision loss in advanced uveitis and can persist or recur even after the ocular inflammation has improved or resolved (Ref. Reference Massa81). Other complications seen in late stages of the disease are retinal detachment, optic disc atrophy and phthisis, a term referring to a shrunk non-functional eye (Ref. Reference Selmi82).
Consequently, similar to glaucoma, uveitis has a significant socioeconomic impact, particularly considering its high prevalence among young adults of working age (Ref. Reference Patel4). In fact, 35% of patients with uveitis present with legal blindness or significant vision loss (Ref. Reference Tsirouki83). The standardisation of uveitis nomenclature group classifies the disease as anterior, intermediate, posterior or panuveitis, based on the primary anatomical site of inflammation. Additional descriptors include disease onset, duration and course (Ref. Reference Jabs84). Uveitis can be further classified clinically according to its aetiology, which includes infectious (bacterial, viral, fungal or parasitic), non-infectious and masquerade (neoplastic or non-neoplastic) (Ref. Reference Deschenes85). Non-infectious uveitis (NIU) is the most prevalent type in developed countries and can be categorised as autoimmune, secondary to an underlying systemic condition or idiopathic (Ref. Reference Hassan86). This review will focus on NIU since treatment for the infectious form and masquerade syndromes aims at tackling the pathogen or the underlying condition, respectively.
Clinical symptoms of NIU can differ considerably even within the same category. Anterior uveitis may manifest with pain and redness, particularly when associated with spondyloarthritis/human leucocyte antigen (HLA) B27. It can also present with blurred vision, ocular injection, watering and sensitivity to light. In advanced stages the condition can lead to synechiae, which is when the pupil exhibits an irregular shape because of adhesions between the iris and the cornea or lens (Refs Reference Burkholder and Jabs87, Reference Duplechain88, Reference Prem Senthil89). Symptoms and signs are less evident when the inflammation involves the posterior segment. In this case, blurred vision and floaters are reported in intermediate uveitis, whereas the posterior type additionally presents vision loss and visual disturbances called dysphotopsias (Refs Reference Selmi82, Reference Dunn90). Panuveitis can manifest as a combination of all these symptoms (Ref. Reference Duplechain88).
Pathogenesis and management
Although the pathogenesis of NIU has not been fully elucidated, the influence of genetic and environmental risk factors has been suggested (Ref. Reference Huang and Brown6). Similar to other autoimmune diseases, it is thought that an imbalance between regulatory and inflammatory mechanisms, potentially triggered by trauma or environmental factors, combined with genetic background, drives the manifestation and progression of uveitis. Additionally, the autoimmune response plays an important role in NIU pathophysiology (Refs Reference Hou77, Reference Xu91). The management of NIU aims to control the inflammation and achieve sustained remission in order to avoid ocular complications while minimising potential side effects associated with the therapy (Ref. Reference Thomas25). CS are usually employed as first-line agents for a powerful, short-term anti-inflammatory effect. Once the acute inflammation is controlled, the long-term management of uveitis is challenging and depends on several aspects, including disease severity, association with a systemic condition and patient's particularities (Ref. Reference Ormaechea92). A stepwise approach is typically employed, introducing different immunomodulatory agents, either alone or in combination, to reduce the CS burden and their attendant ocular and systemic adverse events, especially in prolonged use. Patients exhibiting a suboptimal response to the first-line agent are also subjected to a CS-sparing therapy, which is required in a significant number of cases, either for long-term treatment or because of therapeutic failure (Refs Reference Patel4, Reference Hassan86, Reference Airody93). Hence, antimetabolites, calcineurin inhibitors, biologics and less frequently alkylating agents are utilised as second- and third-line agents. However, severe side effects such as glaucoma, cataracts, liver failure and immunosuppression, along with high costs, less reliable efficacy and clinicians' unfamiliarity, limit the use of these drugs. Consequently, CS remain the mainstay therapy for uveitis (Refs Reference Bordet15, Reference Eskandarpour94). Therefore, there is still an unmet need for therapies that offer improved safety profiles, increased efficacy and long-lasting local effects. Extensive research has been conducted, identifying new inflammatory pathways involved in uveitis and enabling the development of promising candidates, including gene therapies. Specifically, AAV-mediated gene therapies promoting local expression of anti-inflammatory factors are advantageous alternatives to current uveitis treatment (Table 3).
Table 3. Preclinical studies using AAV-mediated gene therapies in models of NIU

AAV, adeno-associated virus; vg, vector genome; ACE2, angiotensin-converting enzyme 2; Ang-(1–7), angiotensin-(1–7); CNV, choroidal neovascularisation; EAU, experimental autoimmune uveitis; EIU, endotoxin-induced uveitis; HLA-G, human leucocyte antigen-G; IRBP, interphotoreceptor retinoid-binding protein; Mas, Mas receptor; NF-κB, nuclear factor kappa B; NIU, non-infectious uveitis; Nrf2, nuclear factor erythroid factor 2-related factor 2; RPE, retinal pigment epithelium; scAAV8, self-complementary AAV8; shRNA, short hairpin RNA; smCBA, truncated chimeric cytomegalovirus (CMV) chicken β-actin; TLR7, Toll-like receptor 7; VEGFA, vascular endothelial growth factor A.
AAV-mediated gene therapies
Gene therapies assessed in multiple models of uveitis
Ildefonso et al. developed a therapy attempting to address some of these factors, consisting of a variant of AAV2 encoding a secretable and cell-penetrating form of M013, a protein from the myxoma virus with inflammasome and nuclear factor kappa B (NF-κB) inhibitory properties (Ref. Reference Ildefonso95). The M013 gene was fused to the sequence of Tat peptide from human immunodeficiency virus (HIV) to enable the secreted protein to penetrate nearby cells and exert its effect (Ref. Reference Ildefonso95). The AAV2 variant (quadY-F + T-V) contained four tyrosine-phenylalanine mutations (at positions 272, 444, 500 and 730) and one threonine-valine mutation at position 491. This serotype had previously showed efficient transduction in several retinal cells after intravitreal delivery (Ref. Reference Ildefonso95). In a mouse model of endotoxin-induced uveitis (EIU), intravitreal pre-treatment with the therapy led to reduced inflammatory infiltrate and lower levels of interleukin 1β (IL-1β) in the vitreous compared with sham controls. A subsequent study evaluated the same therapy in the experimental autoimmune uveitis (EAU) model induced by interphotoreceptor retinoid-binding protein (IRBP) peptide in B10.RIII mice (Ref. Reference Ridley38). Animals pre-treated with the therapy intravitreally exhibited improved clinical signs of intraocular inflammation and reduced expression of proinflammatory markers. Safety assessments in healthy controls showed no changes in retinal thickness and function, suggesting the therapy's safety for intraocular delivery (Ref. Reference Ridley38). EAU is a T cell-mediated intraocular inflammation model that resembles the human autoimmune condition and is suitable for testing new therapies for chronic posterior uveitis, a disease that is particularly challenging to treat (Ref. Reference Shome5).
It is important to highlight that investigating gene therapies for uveitis in two distinct experimental models is a suitable approach given the multifactorial nature of the disease and the difficulty in mimicking all aspects of the human condition. In fact, no single model can recapitulate all features of human uveitis; each one is unique and exhibits specific characteristics of the disease (Ref. Reference Shome5). Ridley et al. (Ref. Reference Ridley38) expanded on the findings reported in the EIU model described by Ildefonso et al. (Ref. Reference Ildefonso95) by employing an experimental model that more accurately recapitulates features of human uveitis and conducted clinical examinations commonly used in human disease monitoring. Nonetheless, a very thorough safety evaluation should indeed be considered to assess the effects of constitutive expression of a viral protein in the eye, not least because it is known that the introduction of exogenous viral proteins in the eye can trigger immune responses and actually exacerbate inflammation (Ref. Reference Mehta, Robbins and Yiu96). Therefore, the use of multiple uveitis models is desirable and might provide additional insights into mechanisms, safety and efficacy and facilitate the screening of potential therapeutic candidates for clinical application.
For instance, Lo et al. explored the specificities of two types of EAU to study the role of Toll-like receptors (TLRs) in uveitis development and propose a gene therapy approach (Ref. Reference Lo37). The EAU induced by IRBP immunisation with adjuvants and the model established by adoptive transfer of IRBP-specific T cells were employed in order to comprehend responses not triggered by adjuvants and select a target involved in both models. A gene therapy consisting of AAV8 encoding short hairpin RNA silencing TLR7 was thus pursued once TLR7 signalling activation led to impairment of the barrier function of retinal pigment epithelium (RPE) cells and exacerbation of the inflammatory response (Ref. Reference Lo37). TLR7 was upregulated in both disease models and subretinal treatment with its agonist led to exacerbation of the inflammatory response in EAU mice (Ref. Reference Lo37). A subretinal injection with this therapy given to mice on the day of uveitis induction resulted in attenuated disease severity in both models 12 days later. This preliminary data confirmed the involvement of TLR7 signalling in EAU development and may support further studies targeting this pathway for uveitis. In addition, the authors drew important conclusions regarding mechanisms implicated in TLR7 signalling in EAU with the proposed therapy. Hence, the study underscores the applicability of AAV-mediated gene delivery both as a therapeutic approach and as a means to enhance our understanding of disease pathogenesis. Moreover, RNA-mediated silencing is a promising approach for ocular diseases and has shown encouraging results in clinical trials (Ref. Reference Kumar97). This strategy, combined with AAV-mediated delivery and expression using cell-specific promoters, may reduce the risk of off-target effects, a common concern in RNA interference, and improve the safety of gene therapy candidates (Ref. Reference Kumar97).
Gene therapies assessed in multiple disease models
Ildefonso et al. reported a different therapeutic strategy and assessed the therapy in multiple disease models (Ref. Reference Ildefonso98). The authors utilised NaIO3-induced RPE oxidative injury and EIU models to investigate the anti-inflammatory and antioxidative properties of a peptide derived from the Nrf2 (Ref. Reference Ildefonso98). The Nrf2-derived peptide was fused to the Tat sequence to promote cell penetration of the expressed protein, which was validated in the study (Ref. Reference Ildefonso98). The secreted and cell-penetrating peptide allows the nuclear translocation of endogenous Nrf2, whose signalling seems to be involved in both neuroprotection effective in glaucoma models and the pathogenesis of uveitis. This peptide sequence was packaged into AAV2 (quadY-F + T-V), the same mutant used in Ildefonso et al. (Ref. Reference Ildefonso95), and intravitreally injected into mice prior to disease induction (Refs Reference Ildefonso95, Reference Ildefonso98). Reduced levels of proinflammatory cytokines and fewer infiltrating cells were reported in animals with acute oxidative stress damage and uveitis, respectively. Additionally, reduced expression of a marker for protein oxidation and improved retinal function were reported in the oxidative stress model, although it did not result in complete protection of RPE cells as showed in morphological analysis (Ref. Reference Ildefonso98). Despite these positive findings, the authors drew attention to the potential risk of ocular infection with continuous inflammatory suppression in the eye, which could be attenuated with a controlled expression of anti-inflammatory factors. Still, by targeting both oxidative stress and inflammation, this therapy affects processes mutually implicated in the pathogenesis of uveitis and glaucoma, as well as other ocular diseases, and could potentially benefit patients with hard-to-treat chronic conditions.
Similarly, Li et al. developed a gene therapy targeting ocular inflammation and choroidal neovascularisation (CNV) and assessed its efficacy in EAU, EIU and laser-induced CNV models (Ref. Reference Li36). The treatment consisted of three inhibitors: one that binds to vascular endothelial growth factor A (VEGFA), the other to C3b/C4b components of complement and a dual inhibitor containing both VEGF and complement binding motifs. The construct was packaged and intravitreally delivered to mice before disease induction. A variant of AAV2 with six mutations (sext Y-F; Y252, 272, 444, 500, 704, 730F) was used for widespread retinal transduction. The therapy ameliorated inflammation and neovascularisation in all tested models, with the dual-acting system promoting better results in EAU and CNV but not in EIU, compared with each inhibitor alone (Ref. Reference Li36). Interestingly, only the combined and the anticomplement alone approaches reduced inflammatory infiltration in EIU, whereas the three strategies improved EAU, highlighting the differences in these experimental mechanisms and supporting the implication of VEGF and the complement cascade in EAU pathogenesis (Ref. Reference Li36). This result is promising, knowing that elevated levels of VEGF have been detected in the vitreous and aqueous humour of patients with different types of uveitis and it could be a causative factor in uveitic macular oedema (Refs Reference Fukunaga99, Reference Goldhardt and Rosen100, Reference Paroli101). In addition, uveitis and uveitic macular oedema can lead to retinal and choroidal neovascularisation, complications usually treated with anti-VEGF therapy (Refs Reference Patel102, Reference Teper103). Therefore, these findings brought attention to what may be possible with multigenic constructs as alternatives for uveitis treatment. The study also reinforced the value of testing new drug candidates in multiple models of uveitis as therapeutic effects seen in EAU were not replicated in EIU.
Gene therapies assessed in a single uveitis model
Kumar et al. also explored the contribution of complement activation to uveitis pathogenesis and suggested AAV2-mediated delivery of soluble CD59 (sCD59) as a potential gene therapy (Ref. Reference Kumar, Cashman and Kumar-Singh104). CD59 prevents the formation of the membrane attack complex (MAC), which seems to be associated with the activation of nucleotide-binding domain-like receptors 3 (NLRP3) inflammasome, involved in several inflammatory conditions. Intravitreal injection of this therapy was given to mice before EAU induction by IRBP. It resulted in attenuated inflammation, with worsened disease severity and progression found in control subjects. However, this therapeutic effect seems to be independent of MAC formation since similar results were not seen in transgenic mice unable to assemble MAC subjected to the same disease model (Ref. Reference Kumar, Cashman and Kumar-Singh104). Still, the gene therapy inhibited MAC deposition and the subsequent NLRP3-mediated production of IL-1β in EAU retinas, which was also seen in transgenic animals. The authors bring attention to the variability of disease severity observed with the animal model employed, which might have contributed to the unexpected outcomes. In addition, they report other mechanisms that might be involved in CD59 anti-inflammatory effects in EAU.
Recent reports from clinical trials conducted by the same group using AAV2-sCD59 (named JNJ-1887) for wet age-related macular degeneration and geographic atrophy (NCT03144999; NCT03585556) are encouraging. These findings may pave the way for its potential application in NIU should further studies prove its safety and efficacy (Ref. Reference Dugel105). It is important to mention that control animals treated with AAV2-GFP exhibited higher clinical scores, albeit not statistically significant, compared with the placebo phosphate buffer-treated group, indicating a possible inflammatory response associated with the vector or the reporter. This finding is in line with reports from the first clinical trial with JNJ-1887 (formerly named HMR59), in which CS therapy was required to control inflammation in a significant number of patients (Ref. Reference Silvent106). In fact, gene therapy-associated uveitis is a condition previously reported in clinical and preclinical studies, with its potential cause attributed to vector dose (Refs Reference Chan107, Reference Tummala108). This dose-dependent inflammation has also been observed in non-ocular gene therapies, representing a common concern in the field since it can affect efficacy and lead to toxicity (Ref. Reference Chan107). This issue is especially relevant when assessing AAV-mediated gene therapies for inflammatory conditions, such as uveitis, whose clinical assessment is likely to be misinterpreted by a vector-related reaction. Therefore, efforts have been made to develop engineered vectors with lower immunogenicity, as well as to establish better immunomodulation protocols and to optimise manufacturing processes to avoid immunogenic impurities (Ref. Reference Hamilton and Wright109). Additionally, engineered vectors with enhanced tropism may allow for lower doses and consequently reduce the risk of vector-related inflammatory reactions (Ref. Reference Gehrke110). Indeed, multiple AAV serotypes with a variety of mutations have been employed in recent investigations for uveitis.
For example, Qiu et al. (Ref. Reference Qiu112) used a tyrosine-capsid mutant (Y733F) AAV8, which has previously demonstrated higher and faster transduction efficiency in murine retinal cells compared with wild-type AAV8 (Refs Reference Petrs-Silva111, Reference Qiu112). The vector has additionally showed efficient transduction in RPE and photoreceptor layers after subretinal delivery in mice (Ref. Reference Qiu112). Hence, the authors chose the subretinal route, which is known to induce a milder inflammatory response compared with the intravitreal (Ref. Reference Ladha113). These vectors were used to deliver the angiotensin-converting enzyme 2 (ACE2) gene, which has shown beneficial effects in models of glaucoma and diabetic retinopathy because of its ability to counteract the deleterious effects of angiotensin II and thereby attenuate inflammation and fibrosis (Refs Reference Qiu112, Reference Dominguez114). Subretinal treatment with the therapy given before EAU induction by IRBP resulted in clinical and functional improvement (Ref. Reference Qiu112). It also decreased the expression of inflammatory cytokines and inhibited pathways involved in the pathological process of EAU. Therefore, the study highlights the importance of the renin–angiotensin system (RAS) in regulating ocular inflammation and provides a novel approach by targeting the ACE2/Ang-(1–7)/Mas protective axis. However, Qiu et al. (Ref. Reference Qiu112) primarily demonstrated the protective effect of the gene therapy administered prior to insult/disease, which is consistent with most studies discussed in the review. Although a protective effect may be applicable for managing recurrent uveitis, an ideal therapeutic approach should additionally guarantee efficacy if the therapy is administered following the onset of the disease. Further investigation addressing the vector's efficiency in ex vivo/in vitro human models seems to be a logical way to assist in documenting the impact of the timing of administration. Hickey et al. reported limited transduction efficiency, mainly restricted to the inner nuclear layer, in degenerated human explants treated with the same vector, AAV8 (Y733F) (Ref. Reference Hickey63). In contrast, better results were seen in healthy explants from primates and in mice with retinal degeneration treated with subretinal and intravitreal injections (Ref. Reference Hickey63). The disparity in AAV transduction efficiency across different species has been discussed in several studies, highlighting the importance of screening vectors in multiple settings (Refs Reference Gonzalez115, Reference Hordeaux116, Reference Li and Samulski117, Reference Tabebordbar118). Moreover, it brings attention to the value of human explants in ocular gene therapy development owing to their resemblance to in vivo conditions and suitability for translational research (Ref. Reference Murali119).
Notably, the studies targeting uveitis discussed thus far in this review did not include an experimental group treated with CS or conventional immunosuppressive drugs as positive controls. It does not affect the scientific contribution of each study, although knowing the performance of such therapies compared with conventional drugs might maximise their clinical prospects. On the other hand, Crabtree et al. tested their developed gene therapy against topical CS. The gene therapy consisted of scAAV8 vectors encoding the codon-optimised human leucocyte antigen-G (HLA-G) isoforms 1 and 5 (Ref. Reference Crabtree34). HLA-G is involved in maintaining the ocular immune privilege and has shown beneficial effects in models of dry eye, corneal inflammation and neovascularisation (Ref. Reference Gilger and Hirsch18). An intravitreal injection containing equal amounts of HLA-G1 and HLA-G5 packaged into scAAV8 was given to rats before EAU induction by IRBP. AAV-mediated expression of HLA-G1/5 led to attenuated inflammation, but dexamethasone-treated animals exhibited better efficacy. However, topical CS treatment was accompanied by severe systemic side effects (Ref. Reference Crabtree34). Therefore, the study did demonstrate the advantage on side effects of long-lasting expression of HLA-G1/5 over standard therapy with multiple instillations of CS in a model of uveitis. Although the biodistribution study did not suggest systemic expression of HLA-G1/5, even with neutralising antibodies (Nabs) against AAV8 found in the serum, a more targeted and controlled production would warrant additional safety for this therapy given the tumorigenic potential of HLA-G proteins (Ref. Reference Zhang120). Also, the use of AAV8 for intravitreal delivery is encouraged because of the lower prevalence of Nabs against this serotype compared with commonly used vectors found in human vitreous (Ref. Reference Andrzejewski121). However, the low retinal tropism of AAV8 through this administration route should be considered in models such as EAU, in which the inflammation involves both the anterior and posterior segments of the eye (Refs Reference Shome5, Reference Han122, Reference Wiley123). Therefore, capsid modifications leading to improved retinal tropism, as reported with tyrosine-to-phenylalanine mutations, may be advantageous in future studies with this therapy (Ref. Reference Petrs-Silva111).
Current progress and future directions
AAV-mediated gene expression may offer a local and long-lasting therapy alternative for uveitis patients, improving patients' adherence and alleviating the treatment burden. It may also allow efficient control of the inflammatory response while it reduces risks of undesirable off-target effects with local delivery. Altogether, the studies reviewed here explored multiple pathways implicated in ocular inflammation (Fig. 2) and undoubtedly proposed biologically credible AAV-mediated gene therapies for NIU. They also addressed a variety of strategies that may be employed in AAV-based platforms to ensure optimised gene delivery and therapeutic effect (Table 4). However, further research assessing these therapies when administered after disease induction is needed to accurately represent efficacy in the practical clinical scenario as well as to improve the understanding of the impact of disease-triggered inflammatory responses on vector's efficiency. Furthermore, given the risk of systemic immunosuppression and opportunistic infections, tight expression control and vector specificity are important features to be considered in such therapies. As a multifactorial disease, uveitis has a complex pathogenesis that is not fully understood. In-depth knowledge of animal models and progresses in ocular immunology may contribute to the identification of additional targets for future gene therapies for uveitis and other ocular inflammatory diseases. The diversity of pathways involved in NIU pathogenesis also encourages the development of dual-acting gene therapies targeting multiple processes. A growing number of clinical trials with gene therapies for ophthalmic conditions is likely to advance our understanding of vector-induced inflammation and facilitate the development of effective strategies to control and prevent it. This would not only positively impact uveitis gene therapy research but could potentially boost the relatively limited number of studies utilising AAV-based platforms for uveitis, ultimately paving the way for clinical translation.

Figure 2. Overview of delivered genes and molecular targets of recent preclinical studies with AAV-mediated gene therapies for the treatment of NIU. AAV, adeno-associated virus; ACE2, angiotensin-converting enzyme 2; ARE, antioxidant response element; GOI, gene of interest; HLA-G, human leucocyte antigen-G; NEP, neprilysin; NF-κB, nuclear factor kappa B; NIU, non-infectious uveitis; Nrf2, nuclear factor erythroid factor 2-related factor 2; RAS, renin–angiotensin system; shRNA, short hairpin RNA; TLR7, Toll-like receptor 7; VEGFA, vascular endothelial growth factor A.
Table 4. Strategies and main outcomes of preclinical studies using AAV-mediated gene therapies in models of uveitis
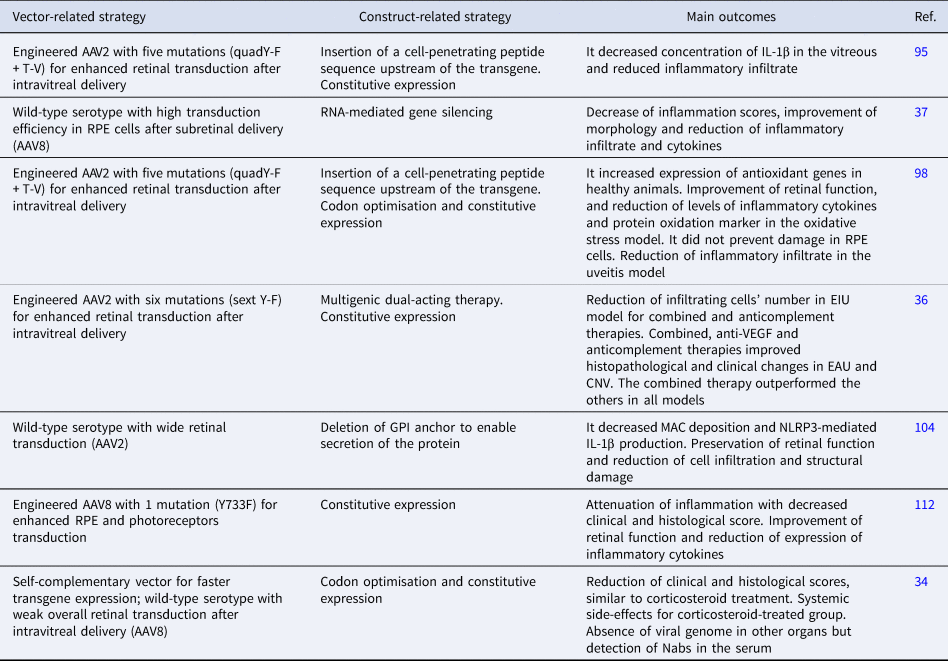
AAV, adeno-associated virus; CNV, choroidal neovascularisation; EAU, experimental autoimmune uveitis; EIU, endotoxin-induced uveitis; F, phenylalanine; IL-1β, interleukin 1β; MAC, membrane attack complex; Nabs, neutralising antibodies; RPE, retinal pigment epithelium; T, threonine; V, valine; VEGF, vascular endothelial growth factor; Y, tyrosine; GPI, glycosylphosphatidylinositol.
Concluding remark
Glaucoma and uveitis are sight-threatening diseases with complex pathogenesis that necessitate improved therapeutic alternatives because of unmet clinical need, compliance challenges and side effects of current treatments. AAV-mediated gene therapies emerge as a promising approach to overcome these challenges, offering a potential solution to the complexities associated with treating these conditions. Clinical trials have reported positive outcomes employing this technology to treat other acquired ocular diseases, which, despite being primarily vascular conditions, share many of the underlying pathological processes of glaucoma and uveitis. Already, multiple strategies and targets have been explored in preclinical studies for glaucoma and uveitis, demonstrating encouraging results regarding safety and efficacy.
In glaucoma, preclinical studies targeting neuroprotection have attempted to overcome limitations regarding long-term expression of the neurotrophic factor BDNF and safety issues from off-target effects of antiapoptotic proteins. In this regard, strategies such as multigenic constructs and cell-specific promoters were employed and have demonstrated positive results. Other approaches explored different pathways providing neuroprotection to RGCs, such as Nrf2 and the tumour suppressor gene MYC signalling, the first controlled by a physiologically induced promoter. Moreover, findings reported with gene therapies affecting other processes involved in the disease mechanism such as oxidative stress, inflammation and the aqueous humour outflow have led to important discussion about treatment timing and use of engineered and self-complementary vectors. These studies have incorporated advanced technologies for gene expression optimisation such as gene editing and drug-inducible systems.
In preclinical studies for uveitis, diverse experimental strategies have highlighted the importance of assessing gene therapies in multiple uveitis and disease models. The first approach was used to investigate therapies targeting the inflammasome, NF-κB- and TLR pathways. In addition, these studies employed relevant therapeutic/delivery strategies, such as engineered vectors, RNA interference and insertion of cell-penetrating sequences. These interventions not only mitigated inflammation under the tested conditions but also contributed to an in-depth understanding of uveitis mechanisms. The utilisation of multiple disease models proved advantageous, especially when studying a gene with dual-acting effects or a multigenic cassette, leading to positive outcomes in combination with vector optimisation. However, concerns about infection susceptibility under continuous immunosuppression emphasise the benefits of controlled expression systems in uveitis gene therapies. Lastly, therapies assessed in a single model of uveitis targeting the complement system, RAS axis or immunotolerance have prompted discussions about viral tropism in different administration routes, gene therapy-associated uveitis, the importance of positive control groups and the necessity to evaluate AAV-based platforms in diverse species, including human settings.
Therefore, careful consideration regarding experimental designs and disease models is crucial to adequately evaluate novel gene therapies for glaucoma and uveitis and enhance the potential for clinical translation. Hence, future directions should focus on further refining AAV-mediated gene delivery and expression, including engineered vectors, optimised constructs and new targets, alongside obtaining an in-depth understanding of disease mechanisms and models.