Abstract
Gliomas are highly heterogeneous in molecular, histology, and microenvironment. However, a classification of gliomas by integrating different tumor microenvironment (TME) components remains unexplored. Based on the enrichment scores of 17 pathways involved in immune, stromal, DNA repair, and nervous system signatures in diffuse gliomas, we performed consensus clustering to uncover novel subtypes of gliomas. Consistently in three glioma datasets (TCGA-glioma, CGGA325, and CGGA301), we identified three subtypes: Stromal-enriched (Str-G), Nerve-enriched (Ner-G), and mixed (Mix-G). Ner-G was charactered by low immune infiltration levels, stromal contents, tumor mutation burden, copy number alterations, DNA repair activity, cell proliferation, epithelial-mesenchymal transformation, stemness, intratumor heterogeneity, androgen receptor expression and EGFR, PTEN, NF1 and MUC16 mutation rates, while high enrichment of neurons and nervous system pathways, and high tumor purity, estrogen receptor expression, IDH1 and CIC mutation rates, temozolomide response rate and overall and disease-free survival rates. In contrast, Str-G displayed contrastive characteristics to Ner-G. Our analysis indicates that the heterogeneity between glioma cells and neurons is lower than that between glioma cells and immune and stromal cells. Furthermore, the abundance of neurons is positively associated with clinical outcomes in gliomas, while the enrichment of immune and stromal cells has a negative association with them. Our classification method provides new insights into the tumor biology of gliomas, as well as clinical implications for the precise management of this disease.
Graphic Abstract









Similar content being viewed by others
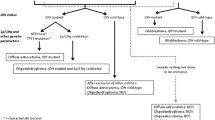
Data availability
All data associated with this study are available within the paper and its supplementary data.
References
Bacolod MD, Talukdar S, Emdad L et al (2016) Immune infiltration, glioma stratification, and therapeutic implications. Transl Cancer Res 5:S652–S656. https://doi.org/10.21037/tcr.2016.10.69
Brat DJ, Verhaak RG, Al-dape KD et al (2015) Comprehensive, integrative genomic analysis of diffuse lower-grade gliomas. N Engl J Med 372:2481–2498. https://doi.org/10.1056/NEJMoa1402121
Verhaak RG, Hoadley KA, Purdom E et al (2010) Integrated genomic analysis identifies clinically relevant subtypes of glioblastoma characterized by abnormalities in PDGFRA, IDH1, EGFR, and NF1. Cancer Cell 17:98–110. https://doi.org/10.1016/j.ccr.2009.12.020
Doucette T, Rao G, Rao A et al (2013) Immune heterogeneity of glioblastoma subtypes: extrapolation from the cancer genome atlas. Cancer Immunol Res 1:112–122. https://doi.org/10.1158/2326-6066.CIR-13-0028
Malta TM, de Souza CF, Sabedot TS et al (2018) Glioma CpG island methylator phenotype (G-CIMP): biological and clinical implications. Neuro Oncol 20:608–620. https://doi.org/10.1093/neuonc/nox183
Osswald M, Jung E, Sahm F et al (2015) Brain tumour cells interconnect to a functional and resistant network. Nature 528:93–98. https://doi.org/10.1038/nature16071
Bagaev A, Kotlov N, Nomie K et al (2021) Conserved pan-cancer microenvironment subtypes predict response to immunotherapy. Cancer Cell 39(845–865):e7. https://doi.org/10.1016/j.ccell.2021.04.014
Feng Q, Li L, Li M et al (2020) Immunological classification of gliomas based on immunogenomic profiling. J Neuroinflammation 17:360. https://doi.org/10.1186/s12974-020-02030-w
Andreotti JP, Silva WN, Costa AC et al (2019) Neural stem cell niche heterogeneity. Semin Cell Dev Biol 95:42–53. https://doi.org/10.1016/j.semcdb.2019.01.005
Rosas-Ballina M, Olofsson PS, Ochani M et al (2011) Acetylcholine-synthesizing T cells relay neural signals in a vagus nerve circuit. Science 334:98–101. https://doi.org/10.1126/science.1209985
Tong N, He Z, Ma Y et al (2021) Tumor associated macrophages, as the dominant immune cells, are an indispensable target for immunologically cold tumor-glioma therapy? Front Cell Dev Biol 9:706286. https://doi.org/10.3389/fcell.2021.706286
Jiang Y, Uhrbom L (2012) On the origin of glioma. Ups J Med Sci 117:113–121. https://doi.org/10.3109/03009734.2012.658976
Del Paggio JC (2018) Immunotherapy: cancer immunotherapy and the value of cure. Nat Rev Clin Oncol 15:268–270. https://doi.org/10.1038/nrclinonc.2018.27
Antunes ARP, Scheyltjens I, Duerinck J et al (2020) Understanding the glioblastoma immune microenvironment as basis for the development of new immunotherapeutic strategies. Elife 9:e52176. https://doi.org/10.7554/eLife.52176
Tomar MS, Kumar A, Srivastava C et al (2021) Elucidating the mechanisms of temozolomide resistance in gliomas and the strategies to overcome the resistance. Biochim Biophys Acta Rev Cancer 1876:188616. https://doi.org/10.1016/j.bbcan.2021.188616
Yoshihara K, Shahmoradgoli M, Martinez E et al (2013) Inferring tumour purity and stromal and immune cell admixture from expression data. Nat Commun 4:2612. https://doi.org/10.1038/ncomms3612
Aran D, Hu Z, Butte AJ (2017) xCell: digitally portraying the tissue cellular heterogeneity landscape. Genome Biol 18:220. https://doi.org/10.1186/s13059-017-1349-1
Kayabolen A, Yilmaz E, Bagci-Onder T (2021) IDH mutations in glioma: double-edged sword in clinical applications? Biomedicines 9:799. https://doi.org/10.3390/biomedicines9070799
Liu Z, Li M, Jiang Z et al (2018) A comprehensive immunologic portrait of triple-negative breast cancer. Transl Oncol 11:311–329. https://doi.org/10.1016/j.tranon.2018.01.011
Sanson M, Marie Y, Paris S et al (2009) Isocitrate dehydrogenase 1 codon 132 mutation is an important prognostic biomarker in gliomas. J Clin Oncol 27:4150–4154. https://doi.org/10.1200/JCO.2009.21.9832
Bunda S, Heir P, Metcalf J et al (2019) CIC protein instability contributes to tumorigenesis in glioblastoma. Nat Commun 10:661. https://doi.org/10.1038/s41467-018-08087-9
Kupp R, Shtayer L, Tien AC et al (2016) Lineage-restricted OLIG2-RTK signaling governs the molecular subtype of glioma stem-like cells. Cell Rep 16:2838–2845. https://doi.org/10.1016/j.celrep.2016.08.040
Fadhlullah SFB, Halim NBA, Yeo JYT et al (2019) Pathogenic mutations in neurofibromin identifies a leucine-rich domain regulating glioma cell invasiveness. Oncogene 38:5367–5380. https://doi.org/10.1038/s41388-019-0809-3
Levi ST, Copeland AR, Nah S et al (2022) Neoantigen identification and response to adoptive cell transfer in anti-PD-1 naive and experienced patients with metastatic melanoma. Clin Cancer Res 28:3042–3052. https://doi.org/10.1158/1078-0432.CCR-21-4499
Knijnenburg TA, Wang L, Zimmermann MT et al (2018) Genomic and molecular landscape of DNA damage repair deficiency across the cancer genome atlas. Cell Rep 23(239–254):e6. https://doi.org/10.1016/j.celrep.2018.03.076
Mermel CH, Schumacher SE, Hill B et al (2011) GISTIC2.0 facilitates sensitive and confident localization of the targets of focal somatic copy-number alteration in human cancers. Genome Biol 12:R41. https://doi.org/10.1186/gb-2011-12-4-r41
Hegi ME, Diserens A, Gorlia T et al (2005) MGMT gene silencing and benefit from temozolomide in glioblastoma. N Engl J Med 352:997–1003. https://doi.org/10.1056/NEJMoa043331
Chen JC, Lee IN, Huang C et al (2019) Valproic acid-induced amphiregulin secretion confers resistance to temozolomide treatment in human glioma cells. BMC Cancer 19:756. https://doi.org/10.1186/s12885-019-5843-6
Fariña-Jerónimo H, de Vera A, Medina L et al (2022) Androgen receptor activity is associated with worse survival in glioblastoma. J Integr Neurosci 21:86. https://doi.org/10.31083/j.jin2103086
Youssef G, Miller JJ (2020) Lower grade gliomas. Curr Neurol Neurosci Rep 20:21. https://doi.org/10.1007/s11910-020-01040-8
Wu F, Wang ZL, Wang KY et al (2020) Classification of diffuse lower-grade glioma based on immunological profiling. Mol Oncol 14:2081–2095. https://doi.org/10.1002/1878-0261.12707
Louis DN, Perry A, Wesseling P et al (2021) The 2021 WHO classification of tumors of the central nervous system: a summary. Neuro Oncol 23:1231–1251. https://doi.org/10.1093/neuonc/noab106
Luoto S, Hermelo I, Vuorinen EM et al (2018) Computational characterization of suppressive immune microenvironments in glioblastoma. Cancer Res 78:5574–5585. https://doi.org/10.1158/0008-5472.CAN-17-3714
Velpula KK, Guda MR, Sahu K et al (2017) Metabolic targeting of EGFRvIII/PDK1 axis in temozolomide resistant glioblastoma. Oncotarget 8:35639–35655. https://doi.org/10.18632/oncotarget.16767
Chen TC, Chuang JY, Ko CY et al (2020) AR ubiquitination induced by the curcumin analog suppresses growth of temozolomide-resistant glioblastoma through disrupting GPX4-Mediated redox homeostasis. Redox Biol 30:101413. https://doi.org/10.1016/j.redox.2019.101413
Kitange GJ, Carlson BL, Schroeder MA et al (2009) Induction of MGMT expression is associated with temozolomide resistance in glioblastoma xenografts. Neuro Oncol 11:281–291. https://doi.org/10.1215/15228517-2008-090
Li M, Zhang Z, Li L et al (2020) An algorithm to quantify intratumor heterogeneity based on alterations of gene expression profiles. Commun Biol 3:505. https://doi.org/10.1038/s42003-020-01230-7
Kanehisa M, Furumichi M, Tanabe M et al (2017) KEGG: new perspectives on genomes, pathways, diseases and drugs. Nucl Acids Res 45:D353–D361. https://doi.org/10.1093/nar/gkw1092
Yoav B, Yosef H (1995) Controlling the false discovery rate: a practical and powerful approach to multiple testing. J R Stat Soc Ser B Stat Methodol 57:289–300. https://doi.org/10.1111/j.2517-6161.1995.tb02031.x
Funding
This work was supported by the China Pharmaceutical University (grant number 3150120001 to XW).
Author information
Authors and Affiliations
Contributions
QF performed the research, data analyses, and manuscript writing. ZD performed the research, data analyses, and manuscript editing. RN preformed data analyses and manuscript editing. XW conceived this study, designed analysis strategies, and wrote the manuscript. All the authors read and approved the final manuscript.
Corresponding author
Ethics declarations
Conflict of interest
The authors declare that they have no competing interests.
Ethics approval and consent to participate
Not applicable.
Consent for publication
Not applicable.
Supplementary Information
Below is the link to the electronic supplementary material.
Rights and permissions
Springer Nature or its licensor (e.g. a society or other partner) holds exclusive rights to this article under a publishing agreement with the author(s) or other rightsholder(s); author self-archiving of the accepted manuscript version of this article is solely governed by the terms of such publishing agreement and applicable law.
About this article
Cite this article
Feng, Q., Dong, Z., Nie, R. et al. Identifying Diffuse Glioma Subtypes Based on Pathway Enrichment Evaluation. Interdiscip Sci Comput Life Sci (2024). https://doi.org/10.1007/s12539-024-00627-w
Received:
Revised:
Accepted:
Published:
DOI: https://doi.org/10.1007/s12539-024-00627-w